Could We Terraform Mars?
Summary
TLDRThis video explores the futuristic and challenging concept of terraforming Mars. It delves into the science behind Marsâ thin atmosphere, the lack of greenhouse gases, and the absence of a magnetic field. The script details potential solutions, from using CO2 from ice caps to large-scale mining operations, solar-powered technology, and even redirecting comets. It also discusses alternative approaches, like building city-sized domes or 'worldhouses,' and touches on broader scientific ideas like magnetic field flipping on Earth. Ultimately, the video imagines a distant future where humanity adapts to and transforms Mars.
Takeaways
- đ Humanityâs future in space is filled with the potential to terraform other planets, starting with Mars.
- đ Marsâ current atmosphere is too thin and cold to support human life, but it was once warmer and had a thicker atmosphere.
- âïž One proposed method to terraform Mars is releasing the CO2 from the planetâs polar ice caps, but this wouldnât be enough to create a sustainable atmosphere.
- đ Mars' lack of a magnetic field, due to its small core, makes it vulnerable to losing any atmosphere it gains to solar winds.
- âïž Extracting CO2 from Marsâ surface or carbonates deep in the crust could increase atmospheric pressure, but would require enormous energy and technology.
- đĄ Terraforming Mars within a generation would require building immense energy infrastructures like solar cells or fusion plants.
- đ Mars has enough water to form some lakes, but not oceans; deep crust sources of water might help.
- đ§Ș The new CO2-oxygen atmosphere would still be toxic to humans, requiring photosynthesizing organisms like algae to convert it into something more breathable.
- đ°ïž Long-term solutions could involve introducing nitrogen or redirecting comets to Mars to bring in new atmospheric materials.
- đïž Instead of full-scale terraforming, paraterraformingâcovering sections of Mars with domes or âworldhousesââmight be a more practical approach for human habitation.
Q & A
What is the main challenge in terraforming Mars?
-The main challenge in terraforming Mars is creating a breathable atmosphere with enough pressure, greenhouse effect, and protection from radiation. Mars' current atmosphere is too thin, lacks sufficient CO2 and nitrogen, and does not protect against harmful cosmic rays.
Why is the lack of a magnetic field on Mars significant for its atmosphere?
-Mars lacks a global magnetic field, which allowed the solar wind to strip away much of its atmosphere over billions of years. Earth's magnetic field protects its atmosphere by deflecting these solar winds, a protection Mars lacks.
Can nuking Mars' polar ice caps help terraform the planet?
-No, nuking Mars' polar ice caps wouldn't release enough CO2 to make a significant difference. The CO2 is buried too deep, and even if all of it were released, it wouldn't be nearly enough to create an atmosphere that resembles Earth's.
What alternative methods are suggested for terraforming Mars?
-Alternatives include mining deep carbonates in Mars' crust, introducing 'super' greenhouse gases like CFCs, or crashing comets into the planet to release nitrogen and water. Another idea is to build massive 'worldhouses'âairtight domes covering large areas of the surface.
How much CO2 would be needed to duplicate Earth's atmospheric pressure on Mars?
-To duplicate Earthâs atmospheric pressure on Mars, weâd need around 10 tons of CO2 per square meter, which would require digging at least 10 meters deep across the entire planet. This translates to processing quadrillions of tons of rock.
Why can't current technology terraform Mars?
-Current technology cannot terraform Mars because the accessible CO2 reserves on the planet are insufficient, and we don't have the energy resources or machinery capable of extracting and processing the necessary materials from Mars' deep crust.
How does the thin Martian atmosphere affect the possibility of liquid water on its surface?
-Marsâ thin atmosphere makes liquid water impossible because even if it were warm enough, water would sublimate directly from ice to gas. The thin atmosphere can't retain the heat necessary to sustain liquid water on the surface.
Could photosynthesis help terraform Mars?
-Yes, photosynthesis could help terraform Mars, specifically by using certain algae, like cyanobacteria, to oxygenate the atmosphere. These organisms are capable of surviving in a CO2-dominated environment and could begin the process of making Mars' atmosphere more Earth-like.
What role could robotic spacecraft play in Mars' terraforming?
-Robotic spacecraft could be used to guide comets from the Kuiper belt to Mars, delivering essential gases like nitrogen and water. A fleet of these spacecraft would nudge comets in the right direction over centuries to contribute to building a breathable atmosphere.
What is a 'worldhouse' and how could it be used on Mars?
-A 'worldhouse' is a concept of creating airtight domes or bubbles on Mars that could cover large areas, providing Earth-like environments inside. This approach would be more feasible in the near-term than terraforming the entire planet, as it requires far less energy and material.
Outlines
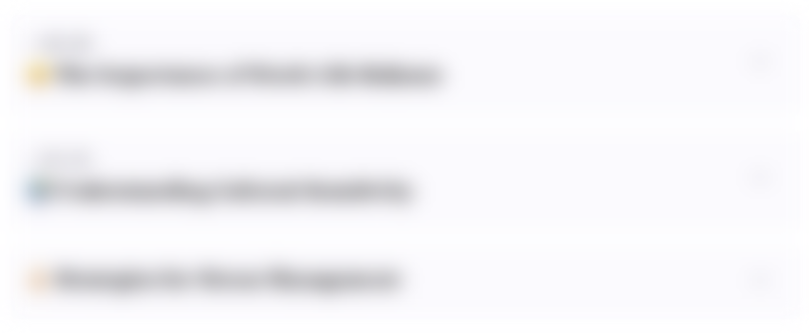
Dieser Bereich ist nur fĂŒr Premium-Benutzer verfĂŒgbar. Bitte fĂŒhren Sie ein Upgrade durch, um auf diesen Abschnitt zuzugreifen.
Upgrade durchfĂŒhrenMindmap
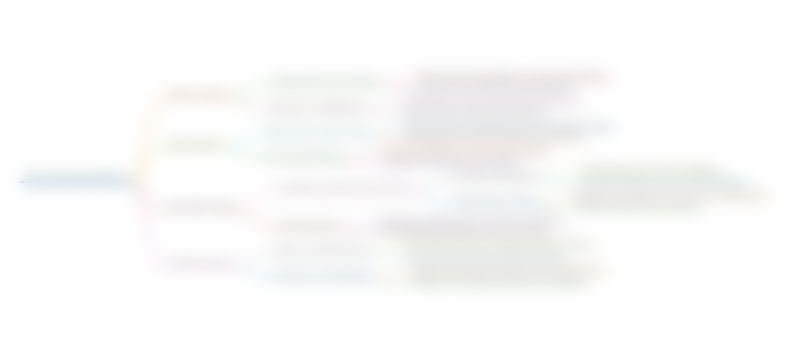
Dieser Bereich ist nur fĂŒr Premium-Benutzer verfĂŒgbar. Bitte fĂŒhren Sie ein Upgrade durch, um auf diesen Abschnitt zuzugreifen.
Upgrade durchfĂŒhrenKeywords
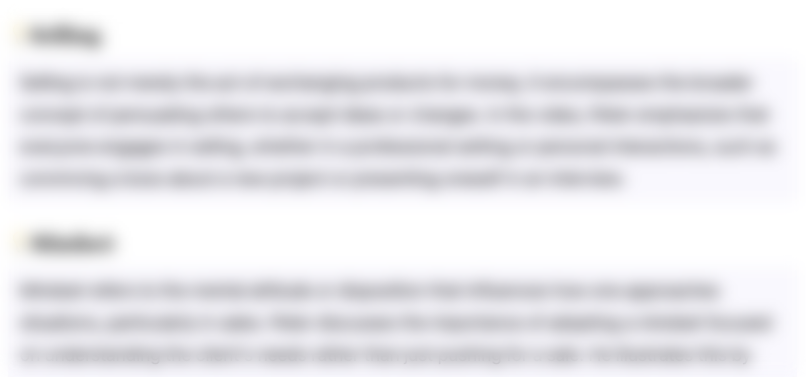
Dieser Bereich ist nur fĂŒr Premium-Benutzer verfĂŒgbar. Bitte fĂŒhren Sie ein Upgrade durch, um auf diesen Abschnitt zuzugreifen.
Upgrade durchfĂŒhrenHighlights
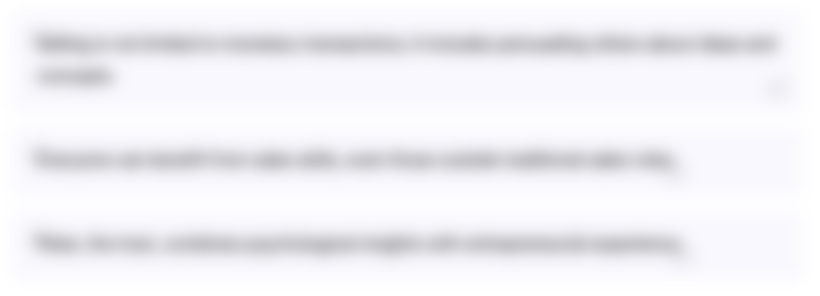
Dieser Bereich ist nur fĂŒr Premium-Benutzer verfĂŒgbar. Bitte fĂŒhren Sie ein Upgrade durch, um auf diesen Abschnitt zuzugreifen.
Upgrade durchfĂŒhrenTranscripts
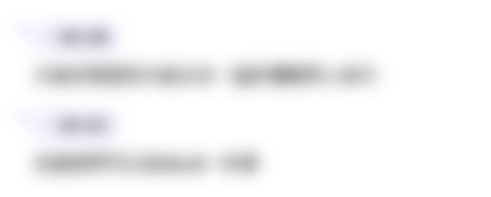
Dieser Bereich ist nur fĂŒr Premium-Benutzer verfĂŒgbar. Bitte fĂŒhren Sie ein Upgrade durch, um auf diesen Abschnitt zuzugreifen.
Upgrade durchfĂŒhren5.0 / 5 (0 votes)