The Only Video Needed to Understand Orbital Mechanics
Summary
TLDRThis video explores the fundamentals of orbital mechanics, explaining why spacecraft must achieve specific velocities and altitudes to maintain orbit. It covers the concepts of kinetic and potential energy, orbital shapes, and maneuvers like prograde, retrograde, and radial burns. Using real-life examples, such as the SpaceX Dragon capsule's rendezvous with the ISS, the video explains how speeding up or slowing down affects a spacecraft's orbit. Viewers learn how manipulating energy and velocity allows for precise navigation in space, and the video emphasizes the counterintuitive nature of orbital mechanics.
Takeaways
- 🚀 Orbits depend on velocity and altitude; high enough horizontal velocity prevents a spacecraft from falling back to Earth.
- 🌍 Rockets need to reach a certain altitude to avoid air resistance and achieve the speed necessary for orbit.
- ⚡ Mechanical energy is the sum of kinetic energy (speed) and potential energy (altitude). Both are key to understanding orbital motion.
- 🎢 Mechanical energy in orbits works like a roller coaster: kinetic energy and potential energy constantly exchange but the total remains the same.
- 🔄 Orbits are usually elliptical, with the closest point called perigee and the farthest point called apogee when orbiting Earth.
- 🔥 Prograde burns (firing engines in the direction of motion) increase velocity and altitude, making the orbit more elliptical.
- ⏪ Retrograde burns (firing in the opposite direction) reduce velocity and lower the altitude on the opposite side of the orbit.
- 📉 Radial burns (firing engines towards or away from Earth) don’t increase velocity but rotate the orbit without significant energy gain.
- 📐 Normal and anti-normal burns change the inclination of the orbit, but are fuel-intensive and used for orbital corrections.
- 🛰️ When trying to rendezvous with the ISS, a retrograde burn is often used to lower altitude and speed up, then a prograde burn is used to circularize the orbit.
Q & A
What is the basic requirement for a spacecraft to stay in orbit?
-A spacecraft needs to achieve sufficient horizontal velocity at a high altitude to stay in orbit. This velocity must counteract the pull of Earth's gravity, allowing the spacecraft to continuously 'fall' around Earth without hitting the surface.
How do kinetic and potential energy contribute to a spacecraft's mechanical energy?
-Mechanical energy is the sum of a spacecraft's kinetic energy (due to its speed) and potential energy (due to its altitude). These two types of energy define its overall mechanical energy in orbit.
Why is air resistance absent in orbit, and why is this important?
-Air resistance is absent in orbit because spacecraft operate at high altitudes where Earth's atmosphere is extremely thin or nonexistent. Without air resistance, spacecraft can maintain the high speeds necessary for orbit without being slowed down.
How does a spacecraft's orbit change when performing a prograde burn?
-A prograde burn increases a spacecraft's velocity and kinetic energy. This causes the spacecraft to gradually climb to a higher altitude, resulting in an elliptical orbit with a larger apoapsis.
What effect does a retrograde burn have on a spacecraft's orbit?
-A retrograde burn decreases a spacecraft's velocity, reducing its kinetic energy. This causes the spacecraft to move closer to Earth on the opposite side of the orbit, lowering the orbit's altitude and making it more elliptical.
Why can't a radial-out burn directly increase the altitude of a spacecraft's orbit?
-A radial-out burn, which points directly away from Earth, does not add horizontal velocity to the orbit. While it can momentarily increase altitude, the spacecraft slows down as a result, causing the opposite side of the orbit to move closer to Earth, effectively rotating the orbit without substantial energy gain.
What is the difference between a normal burn and an anti-normal burn?
-A normal burn changes the inclination of an orbit, causing the spacecraft to rise perpendicular to its current path. An anti-normal burn has the opposite effect, lowering the inclination. These burns rotate the orbit relative to the planet and are fuel-intensive.
What happens to a spacecraft's orbit after a retrograde burn during a rendezvous with another spacecraft?
-During a rendezvous, a retrograde burn decreases the spacecraft's velocity, causing it to descend to a lower orbit and move faster relative to the other spacecraft, helping to close the gap between them.
Why does increasing altitude result in a slower orbital speed?
-As a spacecraft climbs to a higher altitude, its potential energy increases while its kinetic energy decreases. The trade-off between the two means the spacecraft moves slower in a higher orbit due to the conservation of mechanical energy.
How does the moon's orbit illustrate the relationship between orbital altitude and speed?
-The moon, which orbits Earth at a high altitude, moves much slower than satellites in lower orbits. It takes roughly one month to complete one orbit around Earth due to its higher altitude and the corresponding slower orbital speed.
Outlines
🌍 Understanding Orbital Motion and Energy
The video begins by explaining how orbital mechanics defy our expectations of motion and provides an introduction to the basic concepts required to understand how orbits work. It outlines the importance of being in orbit to remain in space and introduces mechanical energy, which consists of kinetic and potential energy. By giving a spacecraft horizontal velocity, it avoids being pulled back to Earth by gravity, achieving an orbit. The segment also emphasizes the need for altitude to avoid atmospheric resistance and introduces the concept of mechanical energy in relation to kinetic and potential energy.
🎢 Energy Exchange in Orbits and Elliptical Paths
This section explains how kinetic and potential energy constantly exchange during motion in an orbit. Using a roller coaster as an analogy, it shows how rising away from Earth decreases kinetic energy while increasing gravitational potential energy, and vice versa. A perfectly circular orbit maintains constant energy, but most orbits are elliptical, with two critical points: the closest (perigee) and the farthest (apogee) distances from Earth. The video introduces these terms and touches on the complexity of manipulating orbits in terms of size, shape, and angle.
🚀 Prograde and Retrograde Burns Explained
This part explains the two most common orbital maneuvers: prograde and retrograde burns. A prograde burn, where engines are fired in the direction of motion, increases velocity but not altitude immediately. Over time, it leads to an elliptical orbit as the spacecraft moves farther from Earth. The opposite maneuver, a retrograde burn, decreases kinetic energy and brings the spacecraft closer to Earth. Both burns are key in controlling altitude and orbital shape, manipulating how a spacecraft behaves within its orbit.
🔄 Radial Burns and Their Effects on Orbit
The focus here is on radial burns, another type of orbital maneuver. A radial out burn is done by firing the engines away from Earth, which temporarily resists gravity and alters the orbit, but causes the spacecraft to slow down. This results in the opposite side of the orbit moving closer to Earth. A radial in burn, where engines fire towards Earth, has a similar effect but in reverse. These burns rotate the orbit without significantly changing the spacecraft's energy levels, emphasizing their limited practical use in altering orbits.
📐 Inclination Changes with Normal Burns
The video discusses normal and anti-normal burns, maneuvers that are perpendicular to the direction of motion and used to change the inclination of an orbit. These burns are fuel-intensive and primarily used for fine-tuning the alignment of orbits, such as when matching the orbit of another spacecraft. Inclination changes are critical when aligning with specific targets, and the video emphasizes their cost in terms of fuel consumption.
🛰 Navigating Orbits with Real-life Examples
The final section applies the learned concepts to a real-world scenario: a SpaceX Dragon capsule rendezvousing with the ISS. The video explains how prograde and retrograde burns affect the spacecraft's orbital altitude and speed relative to the ISS. By firing engines in different directions, the spacecraft adjusts its orbit to align with the ISS, demonstrating how these burns are used in practical space navigation. The video concludes with the importance of circularizing the orbit to match the ISS’s orbit, closing with an invitation to subscribe.
Mindmap
Keywords
💡Orbit
💡Kinetic Energy
💡Potential Energy
💡Prograde Burn
💡Retrograde Burn
💡Elliptical Orbit
💡Apoapsis/Apogee
💡Periapsis/Perigee
💡Mechanical Energy
💡Inclination Change
Highlights
Orbital mechanics can defy normal expectations of motion, requiring a deeper understanding of how objects move in space.
To stay in orbit, a spacecraft needs to achieve a balance between horizontal velocity and the pull of gravity.
High velocities required for orbit can only be achieved in the absence of air resistance, hence the need for high altitude.
Mechanical energy is the sum of kinetic and potential energy in a spacecraft, dependent on its speed and altitude.
A roller coaster is a good analogy for mechanical energy exchange between kinetic and potential energy.
Orbits are generally elliptical, with periapsis (closest point) and apoapsis (furthest point) affecting energy distribution.
Prograde and retrograde burns are common maneuvers to change a spacecraft's orbit, with prograde increasing velocity and retrograde decreasing it.
A prograde burn increases kinetic energy and altitude, making the orbit more elliptical.
A retrograde burn decreases kinetic energy, lowering the spacecraft's altitude on the opposite side of the orbit.
Radial out burns resist gravity but don't provide substantial energy gain, only causing the orbit to rotate.
Normal and anti-normal burns adjust the spacecraft's inclination, rotating its orbit and altering its angle of motion.
Spacecraft rendezvous with the ISS involves intricate orbit matching, using burns to adjust speed and position.
Speeding up a spacecraft can cause it to move into a higher orbit, but paradoxically slow down its orbital velocity.
Slowing down a spacecraft can bring it into a lower orbit, allowing it to approach a faster-moving object like the ISS.
Final orbital maneuvers require circularization to match the target's orbit and complete the rendezvous.
Transcripts
[Music]
orbital mechanics can defy our normal
expectations of motion
and in this animation we'll see how
but to understand how let's first review
a few things
why we need to go into an orbit to stay
in space
what mechanical energy is and we'll also
learn all about orbital Maneuvers we
will then look at why when navigating in
an orbit we need to slow down our
spacecraft to move faster and speed up
to go slower
first of all what makes up an orbit is
it just how far we are from Earth's
surface well if we place the SpaceX crew
Dragon capsule hundreds of kilometers
above Earth with no velocity gravity
represented by the blue arrow will pull
on it and will follow the trajectory of
this blue line and fall out of the sky
towards Earth but if we give it some
horizontal velocity represented by the
red arrow the trajectory begins to
change and if you move fast enough
horizontally the spacecraft will never
hit Earth
these high velocities that are required
to achieve orbit can only be
accomplished with the absence of air
resistance so therefore the rocket has
to have a high enough altitude to be out
of the dense atmosphere
this speed and altitude of the
spacecraft gives the spacecraft kinetic
and potential energy
kinetic energy from how fast it's going
and potential energy from how high it is
or its altitude
these two forms of energy added together
make up mechanical energy and can be
calculated through this formula
a great illustration of mechanical
energy is a roller coaster
a roller coaster train will have
constant mechanical energy but will
continually exchange kinetic energy for
potential energy and vice versa
depending on where it is on the track
as it rises away from Earth it slows
down lowering its kinetic energy but
increasing its gravitational potential
energy
and as it falls to Earth the opposite is
true
a perfectly circular orbit will have a
constant kinetic and potential energy
throughout each orbital period if the
orbiting altitude is constant
but orbits are never perfectly circular
meaning they are elliptic
in elliptical orbit will have a point
that is closest to its orbiting body
which is referred to as the periopsis
and a point where the orbital height is
the furthest from its orbiting body
which is referred to as the apoapsis
when talking about a spacecraft orbiting
Earth these two points are referred to
as the perigee and apogee
manipulating the size shape and angle of
your orbit makes up a large portion of
understanding orbital mechanics
let's briefly review different ways of
manipulating orbits to ultimately
understand why we need to slow down our
spacecraft to go faster and speed up to
go slower
the most common Burns that are used are
the pro-grade and retrograde Burns
pro-grid and retrograde burns are Burns
whose burn vectors are parallel with the
direction of motion let's look at a
specific example of each first the
prograde burn the prograde burn is when
you fire your engines into the direction
you came from a pro-grade burn will
increase your velocity or kinetic energy
at that specific point but not your
altitude or potential energy
but as you continue in your orbit with
higher kinetic energy your inertia will
cause your outward centrifugal force to
be higher than that of gravity resulting
in you to slowly fall further and
further from Earth as you climb in
altitude
your orbit will now be elliptic and look
like this
now let's look at that again
you're added inertia and centrifugal
force is now a little stronger than
before and this causes you to resist
gravity a little more resulting in you
to fall away from Earth
[Music]
if we were to fire our engines in the
exact opposite direction this would be a
retrograde burn and it is very similar
to a pro-grade burn but in the exact
opposite direction
it therefore subtracts kinetic energy
from the orbit at that point of where
you fired your engines and this will
affect the altitude of the opposite side
of your orbit bringing it closer to
Earth
now if you want to increase your Orbit's
altitude can't you just fire your
engines at Earth instead of a pro-grid
burn
well let's do it and see what happens
this burn is known as a radial out burn
a radial out burn will result in your
spacecraft to resist the effects of
gravity to an extent
maintaining a more straight trajectory
throughout the burn but because there's
no velocity added to your orbit as you
raise your altitude from Earth you slow
down this will pull the opposite side of
your orbit towards Earth
the result is that your orbit rotates
about your spacecraft with no
substantial energy gain
a radial inburn where you fire your
Rockets towards space will have the same
effect but in Reverse like this
the last types of burns that can be made
are the normal and anti-normal burns the
normal anti-normal burns are
perpendicular to your direction of
motion as you complete a normal burn
your rocket begins to rise up away from
your initial orbit but because you are
still orbiting the planet your orbit
will slowly rotate with the center of
rotation being the planet you orbit
this change in orbit is referred to as
an inclination change and is a rather
fuel costly maneuver
they are generally only used for
Corrections when matching the orbit of
another spacecraft
now that we've reviewed the basics of
orbital mechanics let's use what we
learned to try to navigate in an orbit
let's look at a real life example a
SpaceX Dragon capsule rendezvousing with
the ISS
say you're in the same orbital path as
the ISS with it just in front of you
intuitively we'll fire our Rockets
behind us to go closer to it a pro-grade
burn
let's see how this affects your orbit
relative to the ISS
this increased kinetic energy will begin
to increase the outward centrifugal
force pulling the spacecraft away from
Earth faster than Earth gravity can pull
back on it
this in return causes the rocket to gain
altitude or potential energy and
therefore lose kinetic energy
much like a roller coaster on the
incline part of a track
this gain and potential energy results
in the spacecraft to lose kinetic energy
our new orbit now has a higher altitude
and therefore a slower average speed
the moon's orbit is a great example of
this higher and slower orbit as it takes
a month to orbit the Earth
if we follow the position of our
spacecraft relative to the ISS it will
rise up above the ISS and fall further
behind as a result of this longer
orbital period
well what if we do just the opposite
let's try firing our Rockets into the
direction we are heading a retrograde
burn slowing us down to see what happens
if we fire our rockets in this direction
we'll decrease our kinetic energy at
that point and our potential energy
Remains the Same
but as we continue to orbit the
decreasing kinetic energy will result in
the spacecraft being pulled to Earth
faster than our inertia pulls it away
this will cause an exchange of energy
from potential to kinetic energy
now if we follow the path of the orbit
relative to the ISS we will begin to
approach it as we pass under it
this only leaves us with one problem we
are only at the correct orbit as the ISS
at one point our apoapsis a last progrid
burn is required to now circularize the
orbit to replicate the orbit of the ISS
thanks for watching and please consider
subscribing
تصفح المزيد من مقاطع الفيديو ذات الصلة
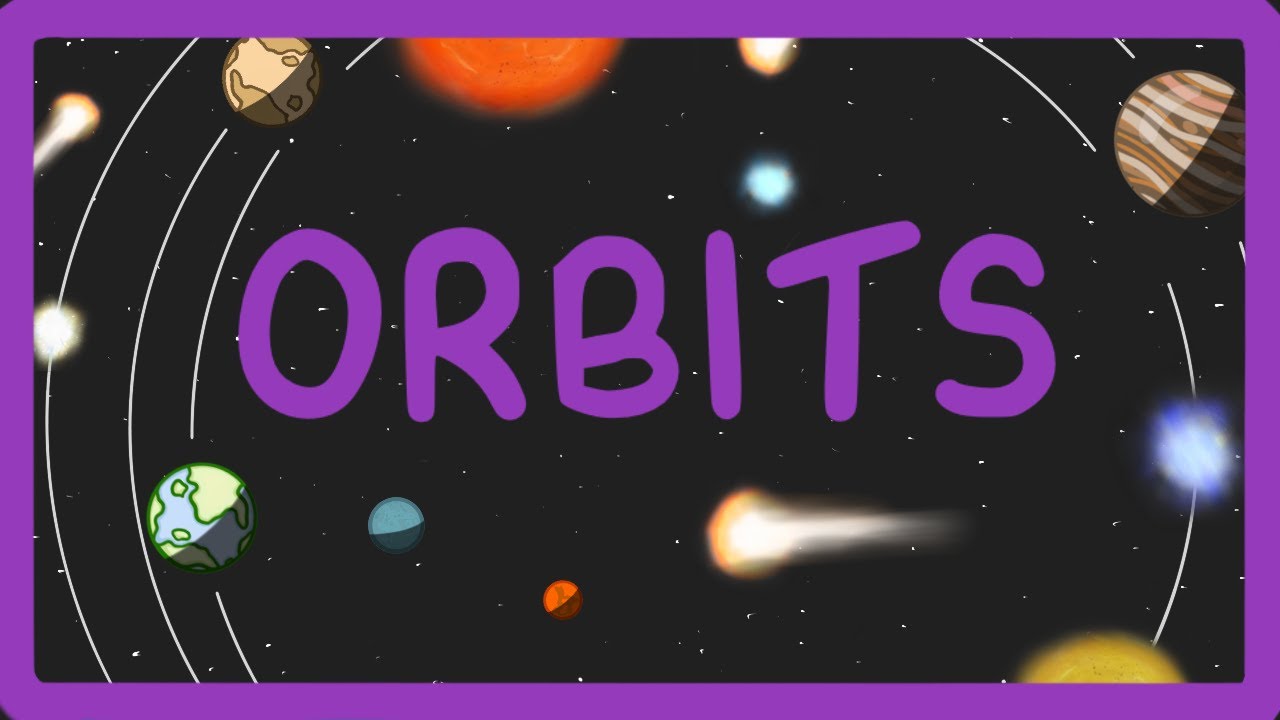
GCSE Physics - What is an Orbit? #86
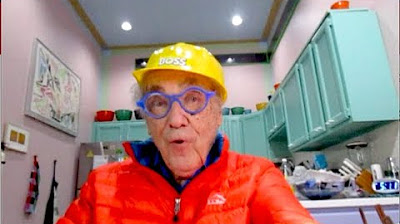
Solution to Moon Problem 211
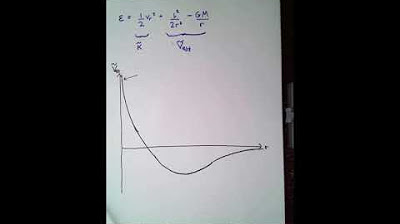
AA-U1-W3-ML1 Physics of Orbits 1 - Energy

Spin-orbit coupling|| Spin-orbit intraction ||fine structure of - H atom|| #spin orbit coupling...
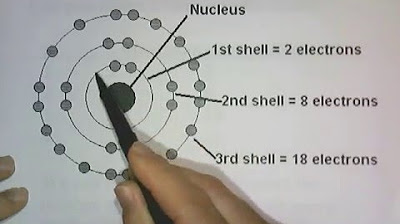
Energy Levels, Energy Sublevels, Orbitals, & Pauli Exclusion Principle

What your teachers (probably) never told you about the parabola, hyperbola, and ellipse
5.0 / 5 (0 votes)