Energy Production in Stars // HSC Physics
Summary
TLDRThis video script delves into the production of energy in stars through nuclear fusion, highlighting Einstein's mass-energy equivalence principle, crucial for understanding the process. It explains the proton-proton chain and the carbon-nitrogen-oxygen cycle, two primary fusion reactions in main sequence stars like our Sun. The script also discusses the triple alpha process in post-main sequence stars, emphasizing the relationship between a star's mass, temperature, and its energy production rate, ultimately affecting its lifespan.
Takeaways
- π Einstein's mass-energy equivalence principle (E=mc^2) is crucial for understanding energy production in stars.
- βοΈ Stars produce energy through nuclear fusion, transforming small amounts of mass into energy.
- π Main sequence stars like the Sun primarily use two types of nuclear fusion: the proton-proton chain (PPC) and the carbon-nitrogen-oxygen (CNO) cycle.
- π« The proton-proton chain involves the fusion of hydrogen nuclei (protons) to form helium, releasing energy in the process.
- βοΈ In the proton-proton chain, four protons are converted into a helium-4 nucleus, with a small mass difference transformed into energy.
- β‘ Using Einstein's equation, the energy produced in the proton-proton chain can be calculated, approximately 27 mega electron volts (MeV) per helium-4 nucleus.
- π The CNO cycle is more complex and involves isotopes of carbon, nitrogen, and oxygen, ultimately producing a helium-4 nucleus.
- π₯ Higher core temperatures in stars enable the CNO cycle by overcoming electrostatic repulsion between heavier nuclei.
- π‘ Heavier stars with higher luminosity and core temperatures tend to use the CNO cycle more than the proton-proton chain.
- π The triple alpha process in post-main sequence stars (like red giants) fuses helium nuclei to form heavier elements like carbon and oxygen.
- πͺ The balance between the outward force from fusion and the inward gravitational force determines the shape and stability of a star.
- π Heavier stars have shorter lifespans due to their higher rate of energy production and faster consumption of mass.
Q & A
What is Einstein's mass-energy equivalence principle?
-Einstein's mass-energy equivalence principle states that energy and mass are interchangeable, governed by the equation E = mcΒ², where energy can be transformed into mass and vice versa. This principle obeys the laws of conservation of energy and mass.
How do stars produce energy through nuclear fusion?
-Stars produce energy via nuclear fusion, a process where small nuclei of elements fuse together to produce a larger nucleus, transforming a small amount of mass into energy.
What are the main types of nuclear fusion reactions in main sequence stars?
-Main sequence stars like our sun produce energy primarily through two types of nuclear fusion reactions: the proton-proton chain (PPC) and the carbon-nitrogen-oxygen cycle (CNO cycle).
What happens in the proton-proton chain?
-In the proton-proton chain, two protons undergo nuclear fusion to produce a hydrogen-2 nucleus, which then fuses with another proton to form a helium-3 nucleus. Two helium-3 nuclei then fuse to produce a helium-4 nucleus and two additional protons.
How is energy produced in the proton-proton chain?
-Energy is produced in the proton-proton chain by converting the mass difference between four protons and the resulting helium-4 nucleus into energy, according to Einstein's mass-energy equivalence principle.
What is the carbon-nitrogen-oxygen (CNO) cycle?
-The CNO cycle is another type of nuclear fusion in main sequence stars, involving a cycle of reactions between isotopes of carbon, nitrogen, and oxygen. At different points in the cycle, four protons are incorporated to form a helium-4 nucleus.
How do core temperature and mass affect the type of nuclear fusion in stars?
-Stars with higher core temperatures and greater mass are more likely to use the CNO cycle for energy production, as the higher temperature helps overcome electrostatic repulsion between heavier nuclei. Lighter stars with lower core temperatures primarily use the proton-proton chain.
What is the triple alpha process?
-The triple alpha process is a nuclear fusion reaction that occurs in post-main sequence stars, such as red giants. It involves the fusion of helium nuclei (alpha particles) to form beryllium-8, which then fuses with another alpha particle to form carbon-12, and further fuses to form oxygen-16.
Why do heavier stars have a greater luminosity?
-Heavier stars have greater luminosity because they need to produce more energy at a higher rate to balance the greater gravitational force exerted on their outer layers. This higher rate of energy production results in greater brightness.
Why do heavier stars have shorter lifespans?
-Heavier stars have shorter lifespans because their higher rate of energy production leads to faster consumption of their mass. Once they run out of mass to convert into energy, their lifespan ends.
Outlines
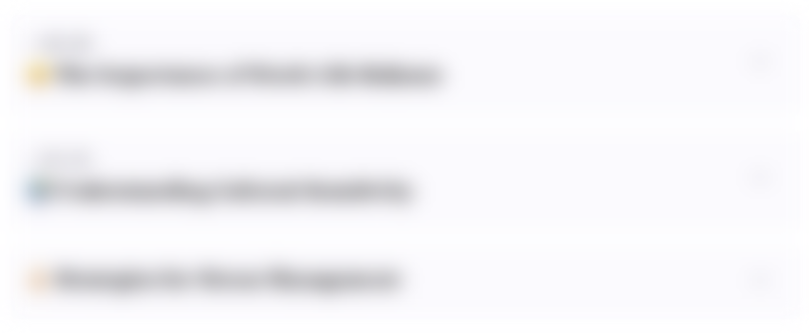
This section is available to paid users only. Please upgrade to access this part.
Upgrade NowMindmap
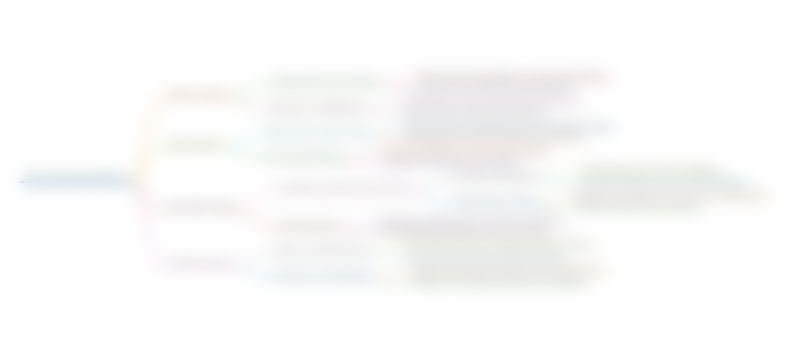
This section is available to paid users only. Please upgrade to access this part.
Upgrade NowKeywords
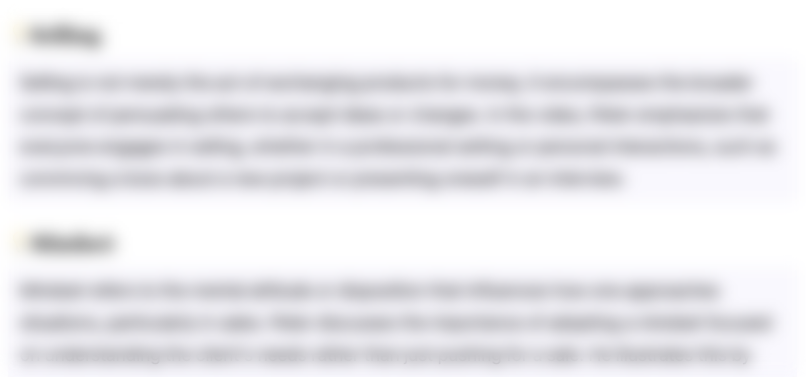
This section is available to paid users only. Please upgrade to access this part.
Upgrade NowHighlights
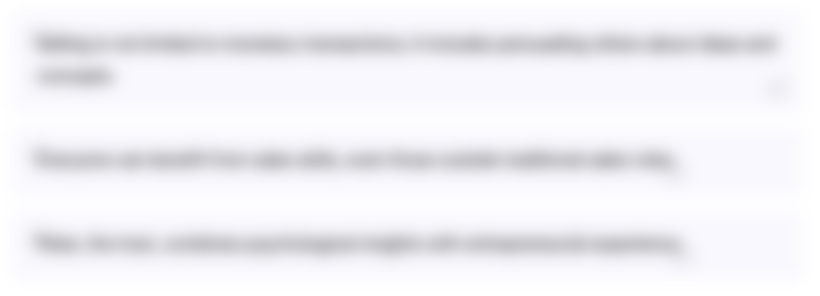
This section is available to paid users only. Please upgrade to access this part.
Upgrade NowTranscripts
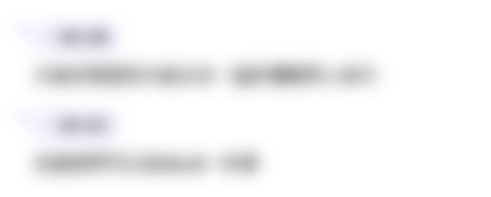
This section is available to paid users only. Please upgrade to access this part.
Upgrade NowBrowse More Related Video

Nuclear Chemistry Part 2 - Fusion and Fission: Crash Course Chemistry #39
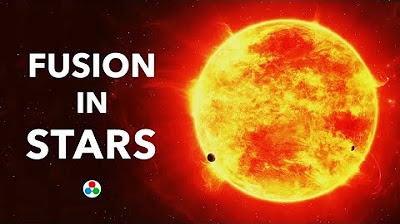
How Stars Turn MATTER into ENERGY | Nuclear Fusion

Nuclear Fusion | Fusion energy explained with Hydrogen atom example | Physics animation video
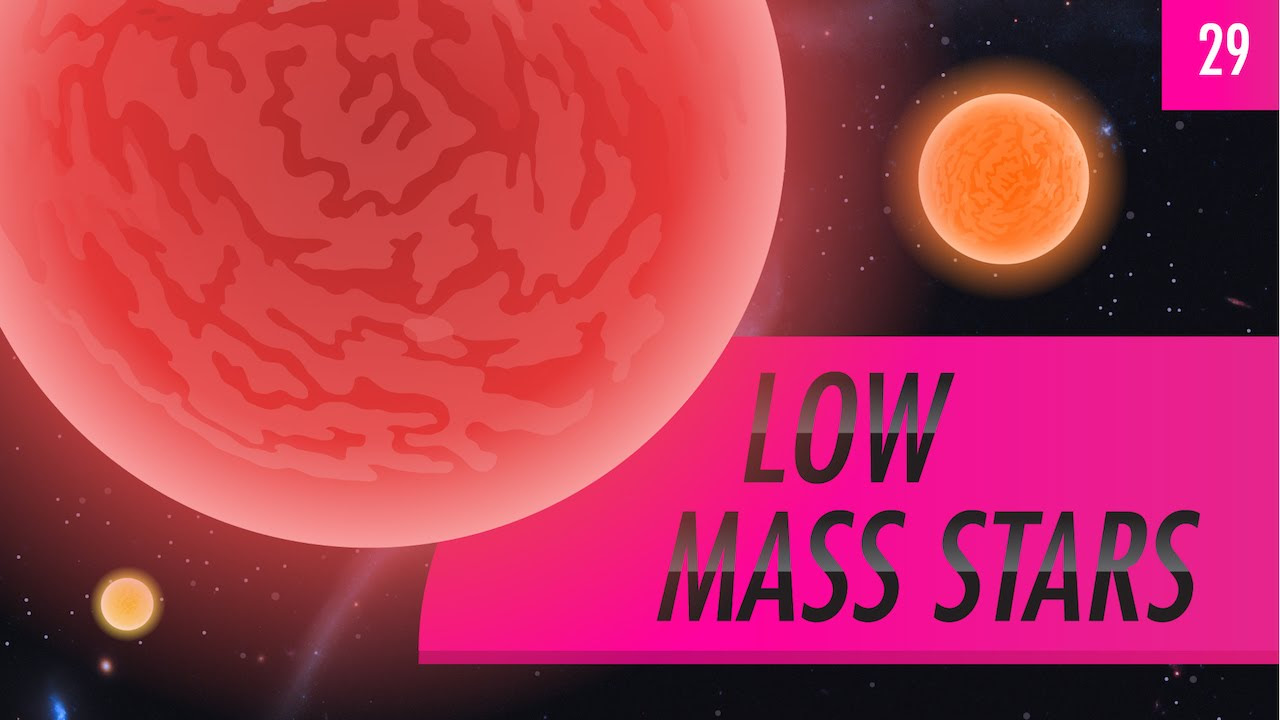
Low Mass Stars: Crash Course Astronomy #29
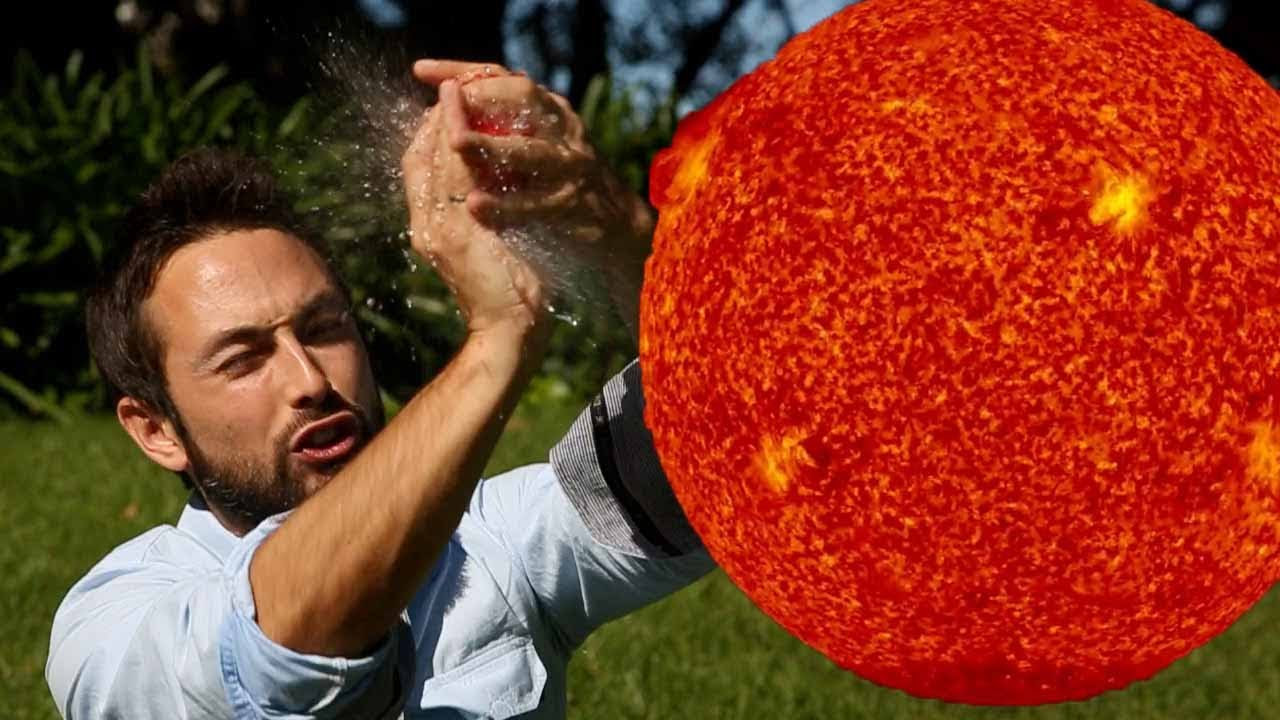
Where Does The Sun Get Its Energy?
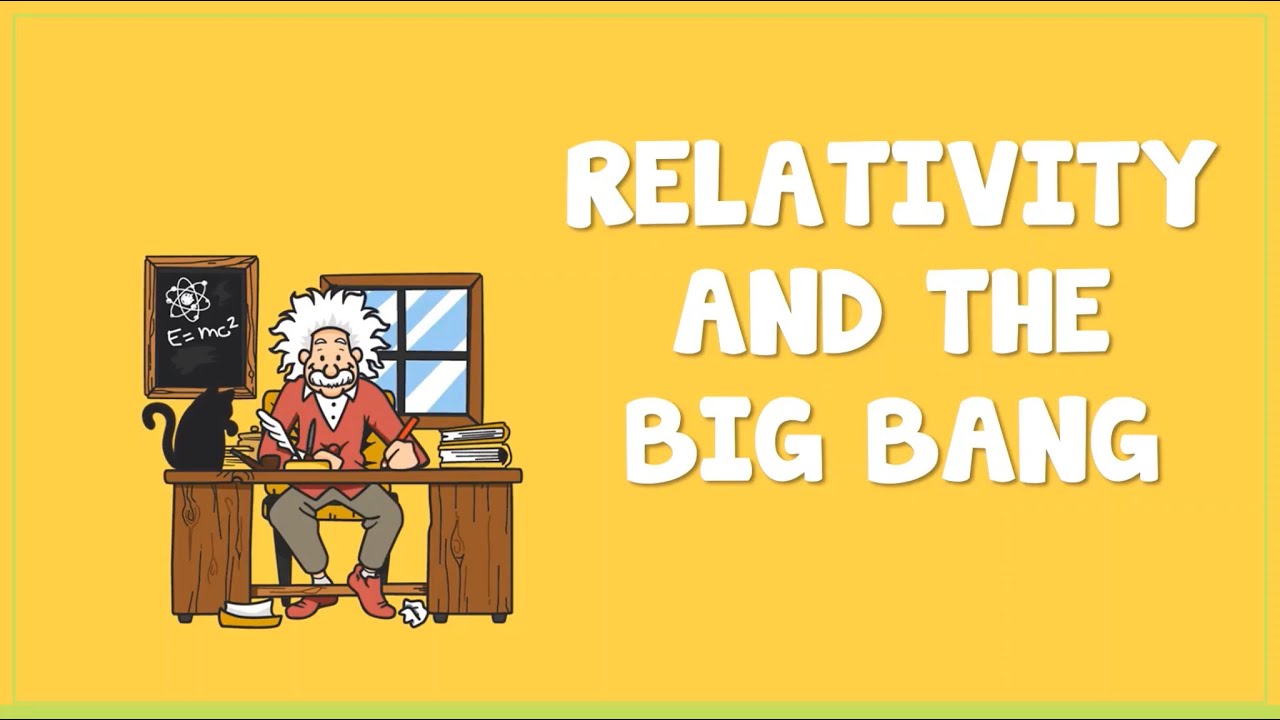
#AghamUnite: Relativity and the Big Bang
5.0 / 5 (0 votes)