MRI Physics | Magnetic Resonance and Spin Echo Sequences - Johns Hopkins Radiology
Summary
TLDRDr. Erin Gomez offers an insightful overview of magnetic resonance imaging (MRI), focusing on the basic spin echo sequence. She explains how protons in the body align with the MRI scanner's magnetic field, creating a net magnetization vector. The video delves into the effects of radio frequency pulses on protons, detailing how they induce changes in longitudinal and transverse magnetization, leading to the generation of signals through free induction decay. It also addresses T1 and T2 relaxation times, T2* effects, and how a 180-degree refocusing pulse combats signal decay, resulting in echo formation crucial for MRI imaging.
Takeaways
- 🧲 MRI Basics: An MRI scanner is essentially a giant magnet that influences protons within the body, aligning them with the external magnetic field (B0).
- 🌀 Proton Behavior: Protons act like tiny bar magnets and usually align randomly but can be influenced by an external magnetic field, leading to a net magnetization vector along the body's z-axis.
- 🔄 Precession and Spin: Protons spin along their axes (precession) at a frequency determined by the Larmor equation, which is dependent on the magnetic field strength and the gyromagnetic ratio of the nucleus.
- 📡 RF Pulses: Externally applied radiofrequency (RF) pulses can alter the alignment of protons, knocking them into a different plane and causing them to precess in sync (transverse magnetization).
- ⏱️ T1 and T2 Times: T1 time is the point where 63% of longitudinal magnetization has recovered, while T2 time is when 63% of transverse magnetization has been lost. These times vary depending on tissue types.
- ⛔ T2 Star Effects: Inhomogeneities in the magnetic field cause protons to dephase rapidly, leading to signal decay known as T2 star effects, which appear as loss of signal in MRI images.
- 🔄 Refocusing Pulses: 180-degree RF pulses can rephase protons that have dephased due to magnetic field inhomogeneity, creating an echo that the MRI scanner reads to generate images.
- 📸 Spin Echo Sequence: In a spin echo sequence, an initial 90-degree pulse is followed by a 180-degree refocusing pulse to produce echoes, which are used to form MRI images.
- ⏰ Timing in MRI: Key timing parameters in MRI sequences include TE (Time to Echo) and TR (Time to Repetition), which define when signals are captured and when sequences are repeated.
- 🖼️ Fast Spin Echo Imaging: This imaging technique uses multiple 180-degree pulses to capture successive echoes, reducing the effects of T2 star decay and enhancing image quality.
Q & A
What is the primary component of an MRI scanner?
-The primary component of an MRI scanner is a giant magnet that generates its own magnetic field, referred to as B0.
How do protons in the human body behave in the presence of an external magnetic field?
-Protons in the human body align with the external magnetic field, with a majority aligning parallel to the primary magnetic field, creating a net magnetization vector.
What is the significance of proton precession?
-Protons precess or spin along their axes in response to the magnetic field, and this precession frequency can be described by the Larmor equation, which relates it to the strength of the magnetic field and the gyromagnetic ratio.
What is the purpose of applying radio frequency (RF) pulses in an MRI scan?
-RF pulses are used to influence the protons by knocking them into an alternate plane and causing them to precess in phase, which changes their longitudinal magnetization.
What is the effect of a 90-degree RF pulse on the net magnetization vector?
-A 90-degree RF pulse causes the net magnetization vector to become perpendicular to its original orientation, eliminating longitudinal magnetization and generating a transverse magnetization vector.
What is the T1 time in the context of MRI?
-The T1 time refers to the point at which 63 percent of the longitudinal magnetization of a proton has been recovered after an RF pulse.
What is the T2 time, and how does it differ from T1 time?
-The T2 time is the point at which 63 percent of the transverse magnetization has been lost. It differs from T1 time in that it measures the loss of transverse magnetization rather than the recovery of longitudinal magnetization.
What are T2 star effects in MRI, and how do they affect image quality?
-T2 star effects are caused by inhomogeneities in the magnetic field, leading to dephasing of proton spins and signal decay. They can appear as diffuse loss of signal or 'black holes' in areas of the image where the magnetic field is particularly distorted.
How can T2 star effects be mitigated during an MRI scan?
-T2 star effects can be mitigated by applying a 180-degree refocusing RF pulse, which temporarily rephases proton precession and produces an echo that can be read by the MRI scanner.
What is the significance of the time to echo (TE) and time to repetition (TR) in MRI sequences?
-The time to echo (TE) is the moment that an echo is produced after a 180-degree refocusing pulse, and the time to repetition (TR) is the time between repetition of sequences. These timings are crucial for controlling the acquisition of MRI signals.
Outlines
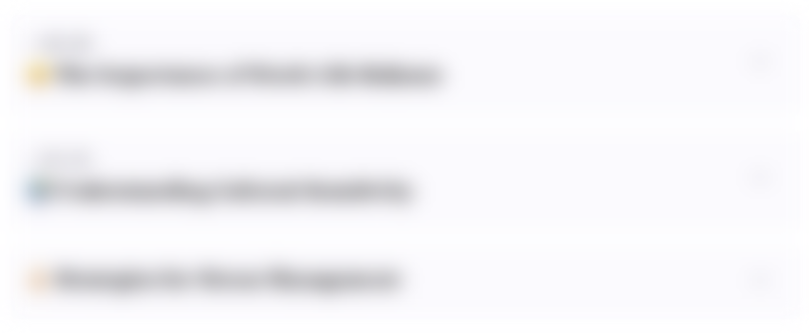
This section is available to paid users only. Please upgrade to access this part.
Upgrade NowMindmap
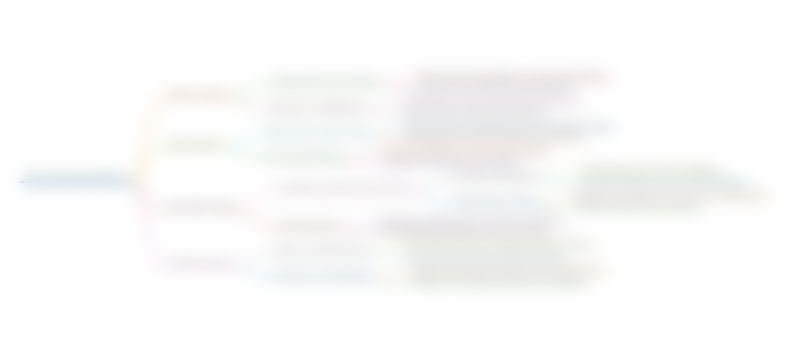
This section is available to paid users only. Please upgrade to access this part.
Upgrade NowKeywords
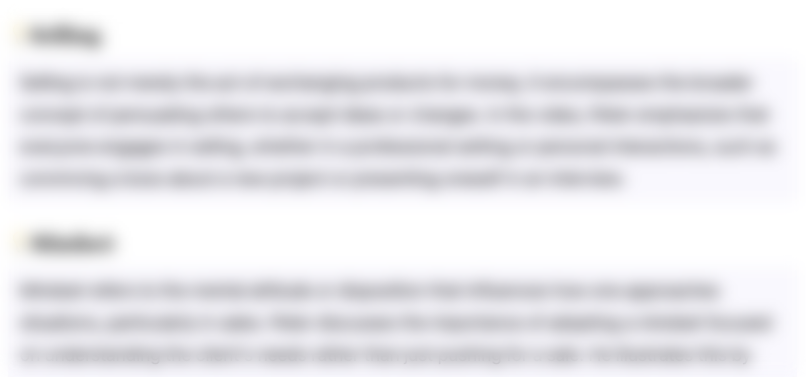
This section is available to paid users only. Please upgrade to access this part.
Upgrade NowHighlights
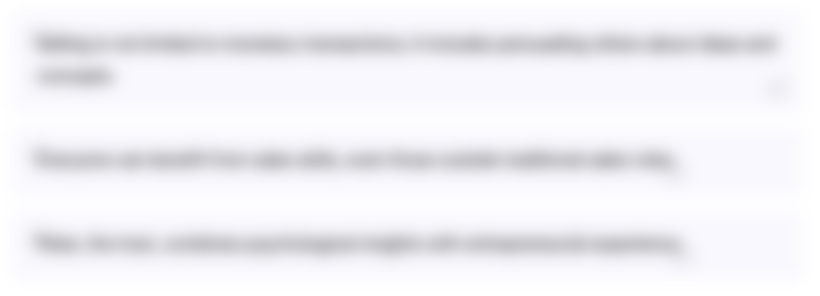
This section is available to paid users only. Please upgrade to access this part.
Upgrade NowTranscripts
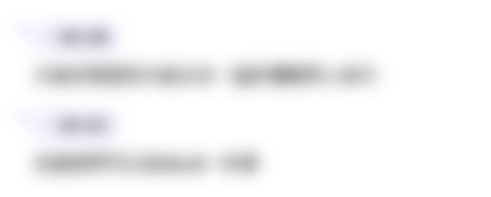
This section is available to paid users only. Please upgrade to access this part.
Upgrade NowBrowse More Related Video

Think Cultural Health Case Study: Cultural and religious beliefs
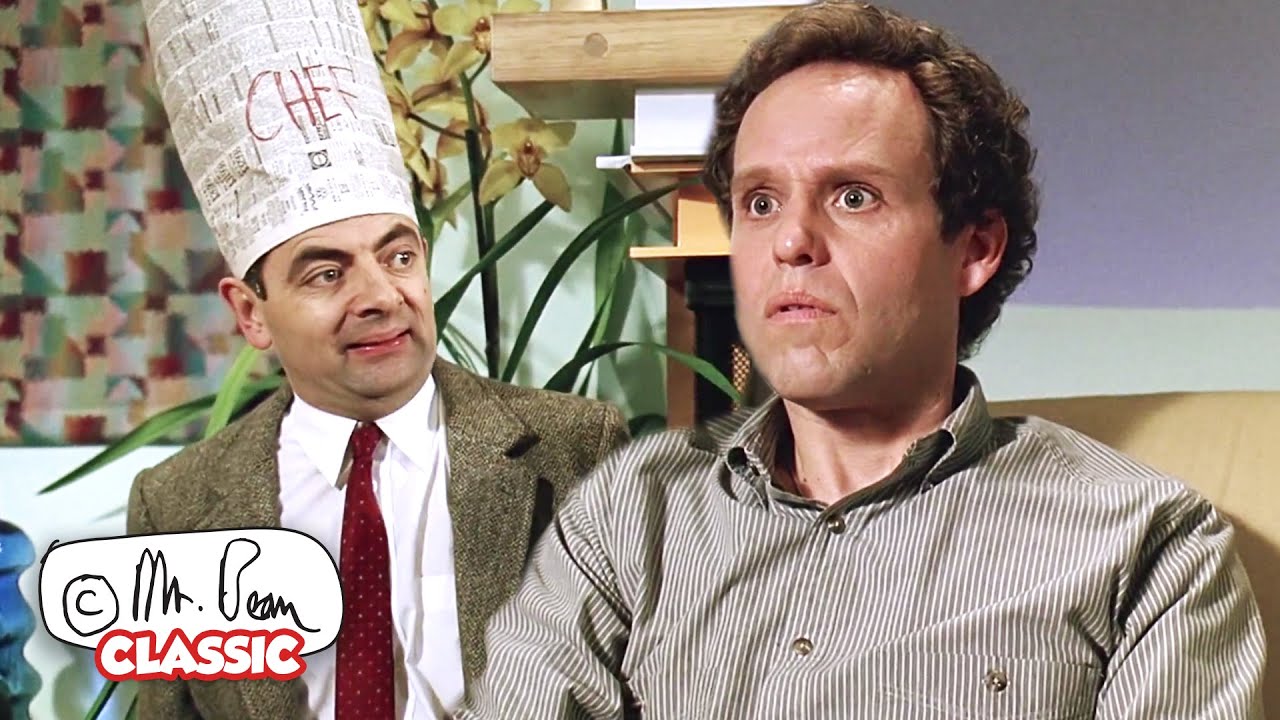
Mr Bean Cooking the CHRISTMAS Dinner | Mr Bean: The Movie | Classic Mr Bean
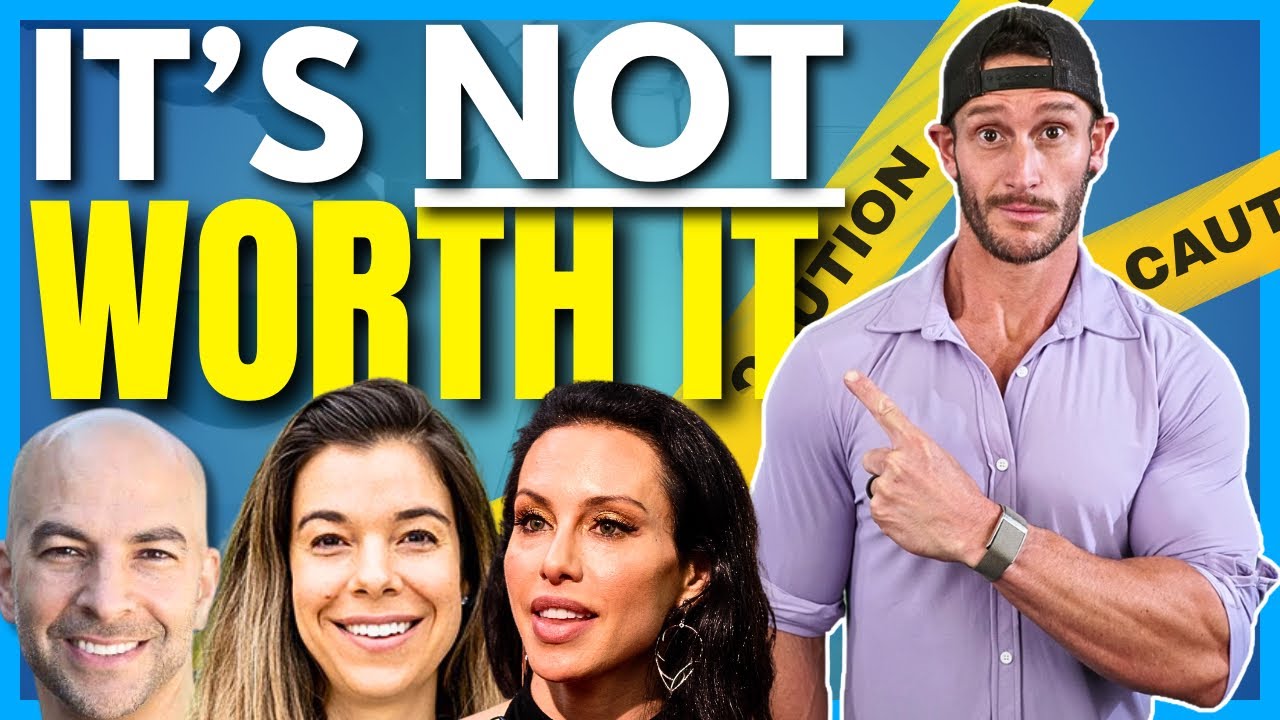
Why Experts are Warning Against Fasting - Dr. Peter Attia, Dr. Rhonda Patrick, Dr. Gabrielle Lyon
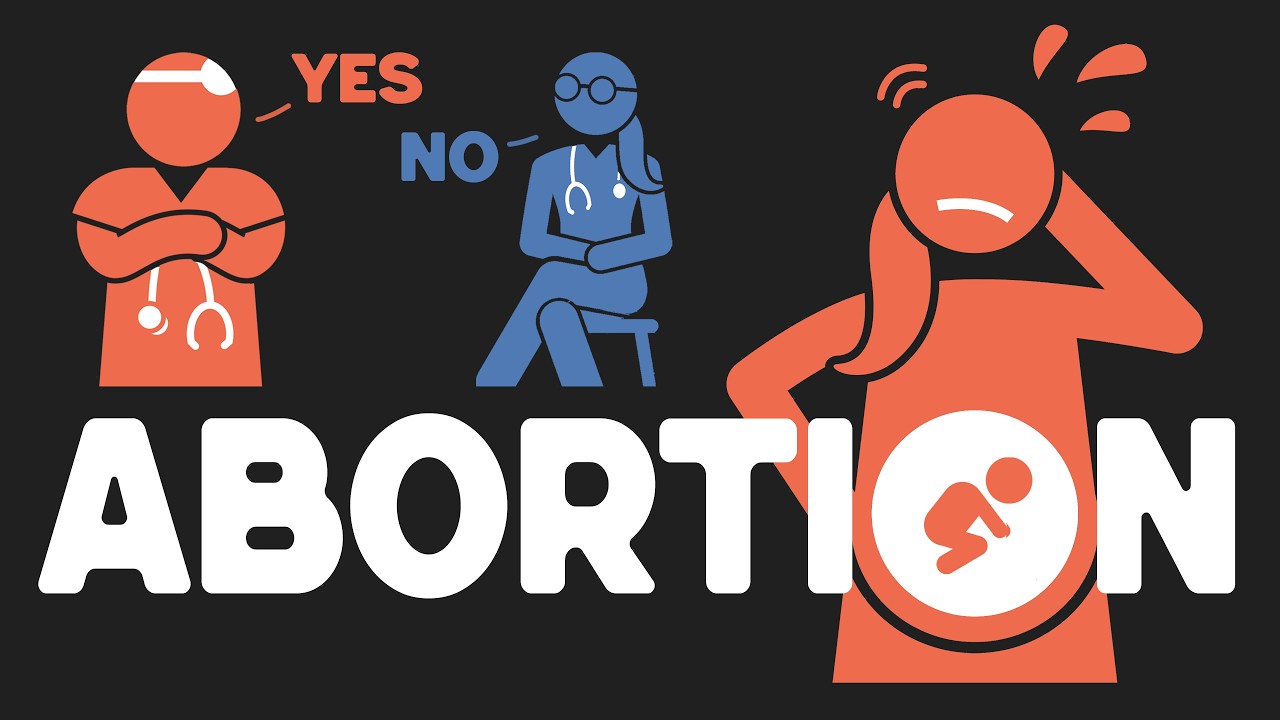
What if AI debated ABORTION?
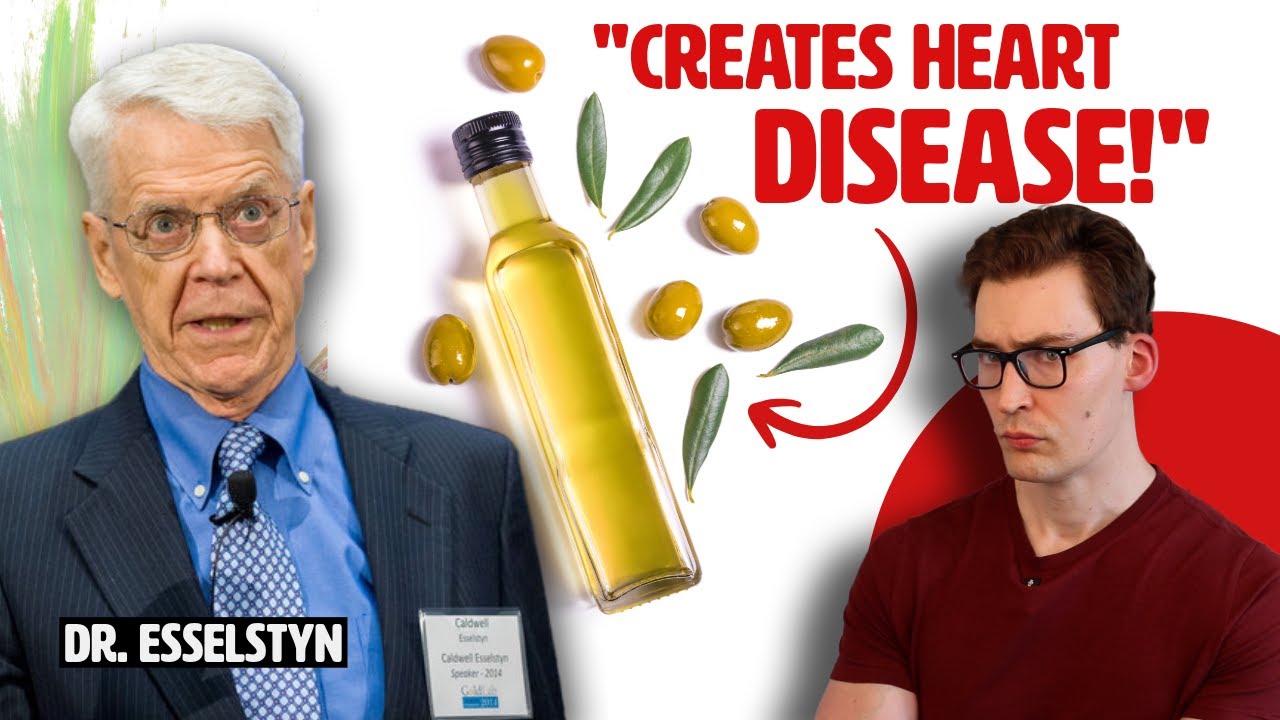
Dr. Esselstyn: “Mediterranean Diet (and Olive Oil) creates Heart Disease!”

Field Study 1-Learning Episode 1: The School Environment
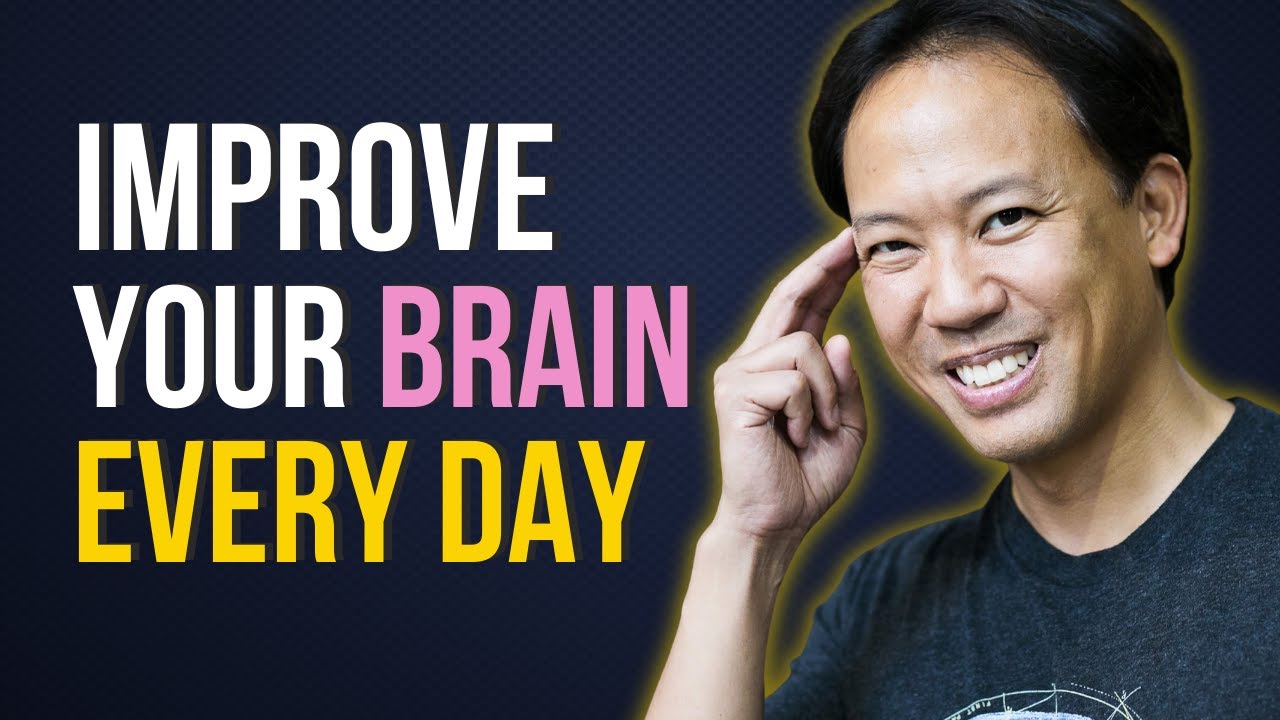
Daily Habits for Better Brain Health | Jim Kwik & Dr. Daniel Amen
5.0 / 5 (0 votes)