Water Potential
Summary
TLDRThis video script delves into the concept of water potential, crucial for understanding osmosis. It explains how water moves into or out of cells based on factors like solute concentration and pressure potential. The script uses visuals to illustrate the differences between hypotonic, isotonic, and hypertonic solutions, and how these affect cell behavior. It also covers the mathematical model of water potential, including the formula for calculating solute potential, emphasizing the importance of understanding how solutes decrease water's ability to move.
Takeaways
- 🌊 Water potential, symbolized by the Greek letter Psi (Ψ), is a measure of the tendency of water to move into or out of a system based on several factors.
- 🔬 The cell membrane's structure, with phospholipids and proteins, plays a crucial role in water movement, especially through aquaporins which facilitate diffusion.
- 🌡 Tonicity (hypotonic, isotonic, hypertonic) describes the concentration of dissolved materials or water relative to a cell, affecting osmosis and water movement.
- 💧 Hypotonic solutions have a higher concentration of water outside the cell, leading to water influx and potential cell swelling.
- 🍇 In hypertonic solutions, the cell loses water to the surrounding solution, causing the cell to shrivel, a process known as plasmolysis in plant cells.
- 🧪 Isotonic solutions have equal concentrations of water inside and outside the cell, resulting in no net movement of water.
- 📈 The change in mass (Δ mass) of a system can be predicted based on the concentration of solutes and the principles of osmosis.
- 🌱 Water potential is modeled mathematically to describe the movement of water in and out of cells, considering factors like pressure and solute potential.
- 📉 Solute potential is always negative, as the presence of solutes in water decreases the water's potential to move, thus lowering the overall water potential.
- 🛡 Pressure potential comes from the rigid cell wall in plant cells, increasing as the cell takes on water and exerts pressure against the wall.
- ⚗️ The solute potential can be calculated using an equation that considers the number of particles a solute dissociates into, the molar concentration, a pressure constant, and temperature in Kelvin.
Q & A
What is the Greek letter symbolized by 'Ψ' used to represent in the context of the video?
-In the context of the video, 'Ψ' (Psi) is used to represent water potential, which describes how water moves into or out of a system based on certain factors.
What are the two main components of a phospholipid molecule found in the cell membrane?
-The two main components of a phospholipid molecule are a negatively charged head made of a phosphate molecule or ion, and neutral or uncharged tails which are fatty acids attached to a glycerol molecule.
How do the properties of the phospholipid bilayer contribute to the structure of the cell membrane?
-The amphiphilic nature of phospholipids, with hydrophilic heads and hydrophobic tails, causes them to orient themselves into a bilayer, with the heads facing the aqueous environment and the tails facing inward, thus forming the cell membrane structure.
What is the role of aquaporins in the cell membrane?
-Aquaporins are integral proteins that facilitate the diffusion of water through the cell membrane, allowing water to travel across it.
What is tonicity and why is it important for understanding water movement?
-Tonicity describes the concentration of dissolved materials or the amount of water in a solution relative to the cell's interior. It is important for understanding water movement because it helps predict the direction of water flow via osmosis, which is from a high concentration to a low concentration.
What happens to a cell in a hypotonic solution?
-In a hypotonic solution, where there is a high amount of water outside the cell and a low amount inside, water will diffuse through the cell membrane into the cell, causing the cell to swell, which in plant cells can lead to turgor and in animal cells can cause the cell to burst.
What is the difference between an isotonic and a hypertonic solution in terms of water movement?
-In an isotonic solution, the amount of water inside the cell is equal to the amount outside, resulting in no net movement of water. In a hypertonic solution, there is less water outside the cell and more inside, causing water to diffuse out of the cell, leading to cell shrinkage or plasmolysis in plant cells.
How can the change in mass (Δmass) be used to predict the direction of water movement in different solutions?
-By knowing the concentrations of solutions, one can predict the change in mass of a system. If the internal solution has a higher concentration than the external, the system will lose water and have a lower Δmass. Conversely, if the internal solution has a lower concentration, it will gain water and have an increased Δmass.
What are the two main components of water potential in a plant cell, as discussed in the video?
-The two main components of water potential in a plant cell, as discussed in the video, are pressure potential and solute potential.
Why is the solute potential always negative?
-The solute potential is always negative because the presence of solutes in water reduces the potential for water movement. Solutes form hydrogen bonds or attractions that limit the ability of water molecules to move freely, thus decreasing the water's potential energy.
How is the solute potential calculated?
-The solute potential is calculated using the equation: Ψs = -(RT/i) * ln(c), where 'i' is the number of particles the solute dissociates into, 'R' is the gas constant, 'T' is the temperature in Kelvin, and 'c' is the molar concentration of the solute.
What is the significance of reaching water potential equilibrium?
-Reaching water potential equilibrium signifies that there is no net movement of water across the cell membrane. This is due to the internal solute potential being balanced by the internal pressure potential, resulting in a stable state for the cell.
Outlines
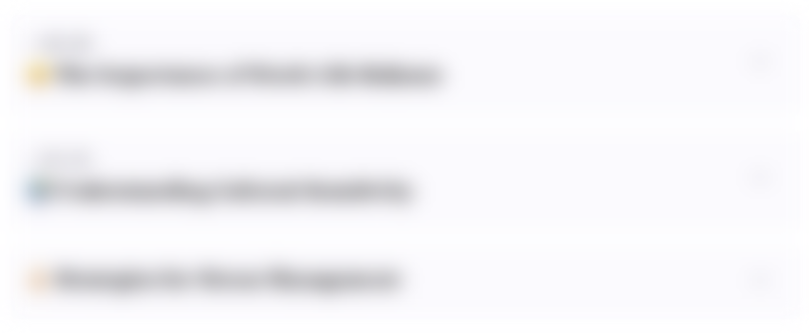
Этот раздел доступен только подписчикам платных тарифов. Пожалуйста, перейдите на платный тариф для доступа.
Перейти на платный тарифMindmap
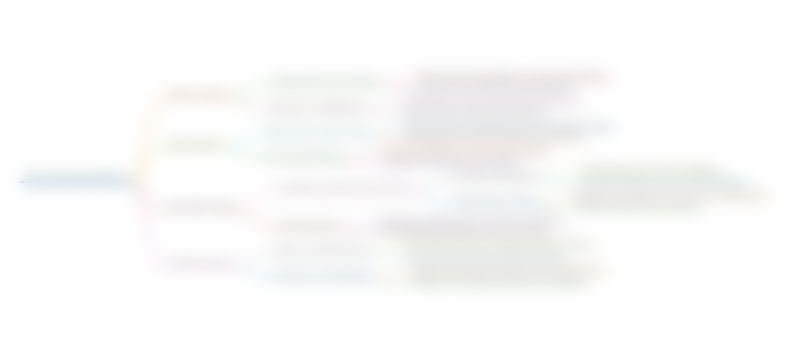
Этот раздел доступен только подписчикам платных тарифов. Пожалуйста, перейдите на платный тариф для доступа.
Перейти на платный тарифKeywords
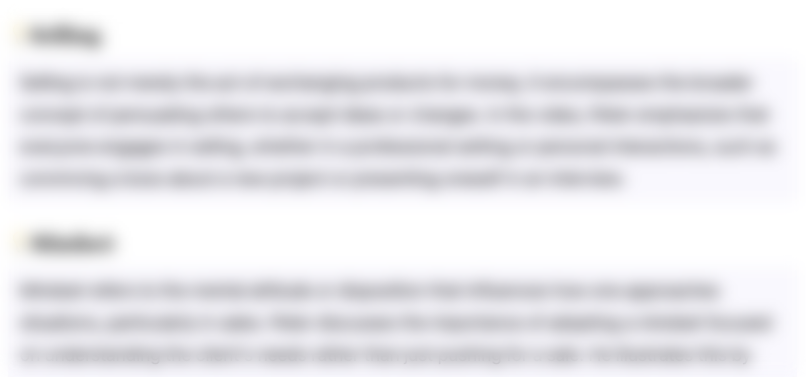
Этот раздел доступен только подписчикам платных тарифов. Пожалуйста, перейдите на платный тариф для доступа.
Перейти на платный тарифHighlights
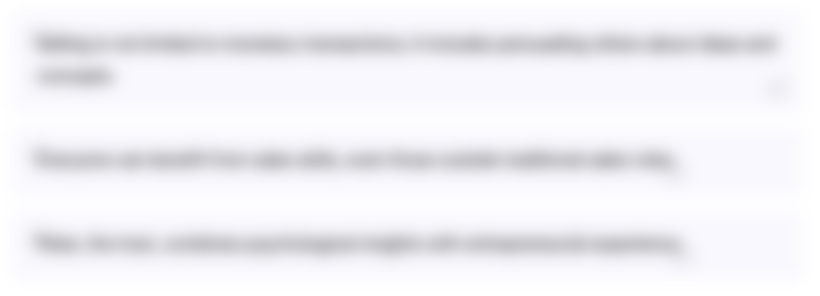
Этот раздел доступен только подписчикам платных тарифов. Пожалуйста, перейдите на платный тариф для доступа.
Перейти на платный тарифTranscripts
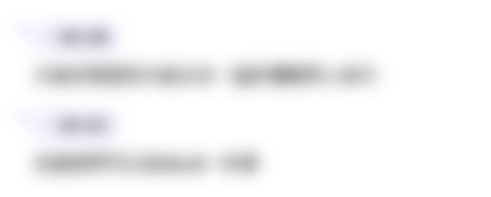
Этот раздел доступен только подписчикам платных тарифов. Пожалуйста, перейдите на платный тариф для доступа.
Перейти на платный тарифПосмотреть больше похожих видео
5.0 / 5 (0 votes)