Laminar boundary layers [Fluid Mechanics #13]
Summary
TLDRThis video delves into the concept of laminar boundary layers in fluid mechanics, focusing on external flows where fluid interacts with a solid surface. It explains how boundary layers form due to viscosity and the no-slip condition at the wall, leading to a velocity gradient near the surface. The video outlines the theoretical approach to analyzing these layers, including assumptions and boundary conditions. It also discusses the significance of boundary layer thickness and how it influences forces like drag and lift. Prandtl and Blasius' solutions for the velocity profile within these layers are highlighted, along with the calculation of wall shear stress and drag force, emphasizing the importance of understanding boundary layers in various applications such as aerodynamics and climate modeling.
Takeaways
- 🌊 The video discusses external flows, which are open on one or more sides and can extend infinitely in at least one direction, unlike enclosed flows.
- 🔍 The focus is on boundary layers, which are regions of velocity gradient near a surface due to viscosity and the no-slip condition at the wall.
- 🎯 The video aims to solve for the velocity and pressure fields in laminar boundary layers, with turbulent boundary layers to be covered in a subsequent video.
- 📚 External flows include boundary layers, jets, wakes, and shear layers, all of which require specific analysis in fluid mechanics.
- 🛠 Assumptions made for analysis include incompressible, steady, two-dimensional, and two-component flow, with no body forces.
- 📉 Observational assumptions are used to simplify the conservation equations, focusing on the gradual growth region of the boundary layer.
- 🧮 The conservation of mass and momentum equations are simplified using these assumptions, leading to a manageable set of equations for boundary layer analysis.
- 📉 Prandtl and Blasius solved the simplified equations, revealing the self-similar shape of the laminar boundary layer's velocity profile.
- 📏 Three methods to measure boundary layer thickness are discussed: disturbance thickness, displacement thickness, and momentum thickness.
- 💨 The video concludes with the calculation of wall shear stress and drag force due to the fluid on the surface, emphasizing the importance of understanding boundary layers in various applications.
Q & A
What is the primary focus of the video?
-The primary focus of the video is on laminar boundary layers, which are regions of lower velocity fluid that grow on an external surface in a flow field due to viscosity.
What are the key differences between enclosed flows and external flows?
-Enclosed flows have boundaries on all sides, while external flows can be open and extend effectively into infinity in at least one direction. External flows often exhibit growth or development in the streamwise direction, leading to more complicated flows.
What is a boundary layer in the context of fluid mechanics?
-A boundary layer is a thin layer of fluid in the immediate vicinity of a bounding surface where the effects of viscosity are significant, resulting in a velocity gradient from the surface to the free-stream velocity.
Why is the no-slip condition important in boundary layer analysis?
-The no-slip condition is important because it states that the fluid velocity at the wall is equal to the wall's velocity, which is crucial for understanding the velocity profile near the surface and the associated shear stress.
What assumptions are typically made when analyzing laminar boundary layers?
-When analyzing laminar boundary layers, it is assumed that the fluid is incompressible, steady, two-dimensional, and two-component. Additionally, it is assumed that there are no body forces, and the flow develops in the x-direction.
How do Prandtl and Blasius contribute to the understanding of boundary layers?
-Prandtl and Blasius are credited with pioneering boundary layer research. They derived and solved the conservation equations for a laminar boundary layer, leading to the understanding of the self-similar shape of the velocity profile.
What are the three main strategies to measure the boundary layer height?
-The three main strategies to measure the boundary layer height are disturbance thickness (δ), displacement thickness (δ*), and momentum thickness (θ), each providing a different perspective on the boundary layer's impact on the flow.
How is the Reynolds number used in boundary layer analysis?
-The Reynolds number is used to characterize the flow and determine when a flow transitions to turbulence. For a smooth flat plate, the transition is estimated to occur around a Reynolds number of five hundred thousand.
What is the significance of the skin friction coefficient in boundary layer analysis?
-The skin friction coefficient (c_f) is a non-dimensional parameter that represents the wall shear stress relative to the flow inertia. It is important for understanding the drag force on a surface due to the fluid flow.
How does the drag force on a surface due to a boundary layer get calculated?
-The drag force on a surface due to a boundary layer is calculated by integrating the shear stress over the surface area. For laminar boundary layers, this is a function of the velocity, plate length, and the Reynolds number based on the plate length.
Outlines
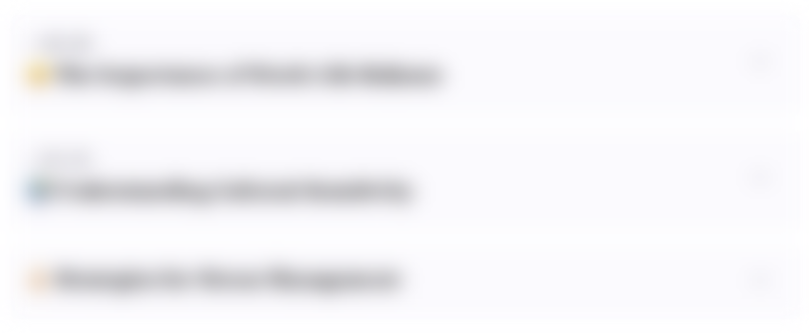
このセクションは有料ユーザー限定です。 アクセスするには、アップグレードをお願いします。
今すぐアップグレードMindmap
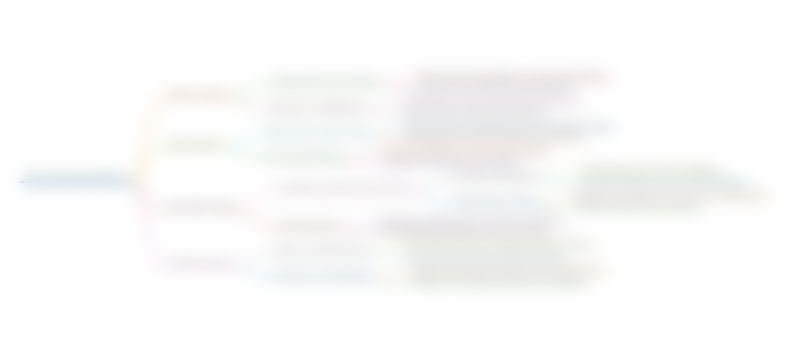
このセクションは有料ユーザー限定です。 アクセスするには、アップグレードをお願いします。
今すぐアップグレードKeywords
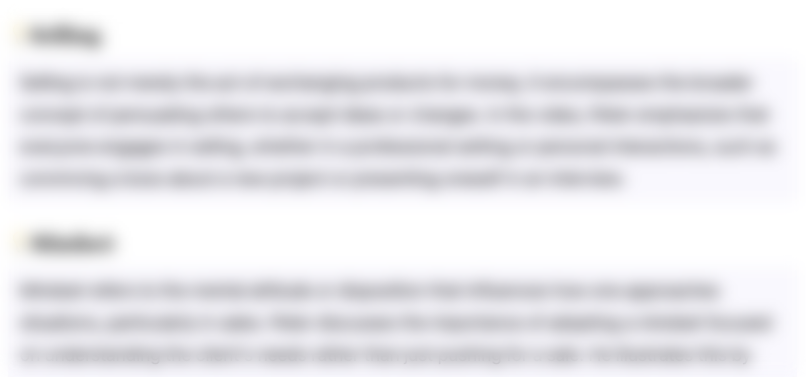
このセクションは有料ユーザー限定です。 アクセスするには、アップグレードをお願いします。
今すぐアップグレードHighlights
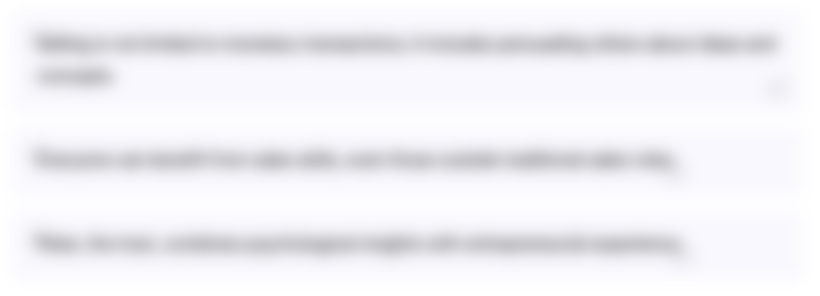
このセクションは有料ユーザー限定です。 アクセスするには、アップグレードをお願いします。
今すぐアップグレードTranscripts
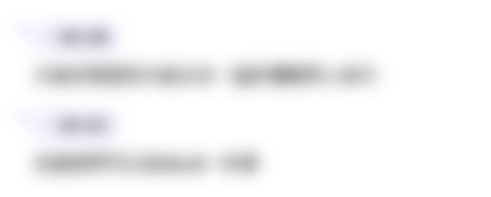
このセクションは有料ユーザー限定です。 アクセスするには、アップグレードをお願いします。
今すぐアップグレード5.0 / 5 (0 votes)