Proteins Structure 4a'
Summary
TLDRThis lecture explores the intricate relationship between genes and proteins, focusing on the translation process and the genetic code. It delves into the composition of RNA, the structure of proteins, and the significance of amino acids. The lecture also discusses the concept of colinearity between genes and proteins, demonstrating how the sequence of a gene directly corresponds to the sequence of a protein. Additionally, it covers the historical experiments that led to the understanding of the genetic code as a triplet code, highlighting the pivotal role of in vitro translation and RNA synthesis in decoding how specific nucleotide sequences dictate amino acid formation.
Takeaways
- 🧬 The lecture introduces the concept of translation in genetics, focusing on the synthesis of proteins from mRNA by ribosomes and other cellular components.
- 📚 mRNA and tRNA constitute over 95% of RNA in a cell, with mRNA making up only 1-2%, indicating their stability and importance in cellular transcription.
- 🔑 The genetic code is the link between the nucleotide sequence of a gene and the amino acid sequence of the protein it encodes.
- 🌟 Proteins are crucial for an organism's phenotype, acting as enzymes to catalyze chemical reactions and forming structural components of cells.
- 🔍 Understanding protein structure is essential for comprehending genes, as proteins are encoded by genes and reflect the information they contain.
- 📝 Amino acids, the monomer subunits of proteins, are polymerized into polypeptides through peptide bonds, forming the primary structure of proteins.
- 🌀 The secondary structure of proteins, such as alpha-helices and beta-sheets, arises from hydrogen bonding between amino acids, contributing to the protein's overall shape.
- 🧬 Tertiary structure refers to the three-dimensional conformation of a single polypeptide chain, influenced by its secondary structures and amino acid interactions.
- 🤝 Quinary structure describes the arrangement of multiple polypeptide chains in a protein complex, held together by various weak bonds.
- 🔄 The concept of colinearity between genes and proteins was demonstrated by Charles Yanofsky, showing that the sequence of a gene directly corresponds to the sequence of its protein product.
- 🧬 The genetic code is a triplet code, with three nucleotides specifying a single amino acid, and is non-overlapping, meaning each codon is read individually.
Q & A
What are the main components involved in protein synthesis?
-Protein synthesis involves messenger RNA (mRNA), transfer RNA (tRNA), and ribosomes. mRNA is read by ribosomes, and tRNA brings the appropriate amino acids to the ribosome to generate proteins.
What percentage of RNA in a cell is made up of rRNA and tRNA?
-rRNA and tRNA make up over 95% of RNA in a cell.
How does the stability of rRNA and tRNA compare to mRNA?
-rRNA and tRNA are more stable than mRNA and do not get degraded and turned over as fast.
What is the relationship between the nucleotide sequence of a gene and the amino acid sequence of a protein?
-The genetic code describes the relationship, where the nucleotide sequence of the gene specifies the amino acid sequence of the protein.
What are the 20 different subunits that make up proteins called?
-The 20 different subunits that make up proteins are called amino acids.
What is a polypeptide and how is it formed?
-A polypeptide is a polymer composed of amino acids, also known as peptides. It is formed by peptide bonds created through condensation reactions between the amino group of one amino acid and the carboxy group of another.
What are the four levels of protein structure?
-The four levels of protein structure are primary (linear sequence of amino acids), secondary (regular repeated structures like alpha helix and beta pleated sheets), tertiary (three-dimensional structure of a single polypeptide chain), and quinary (structure of multiple subunit proteins).
What is the significance of the directionality of polypeptides and mRNA?
-The amino terminus of a polypeptide is lined up with the five prime end of the mRNA, and the carboxy terminus with the three prime end, indicating the direction of translation from the mRNA to the protein.
How was the colinearity of genes and proteins first demonstrated?
-Colinearity was first demonstrated by Charles Yanofsky in 1963 through his work with the trpA gene in E. coli. He induced mutations and showed that each mutation created an amino acid substitution in the same order as the mutations in the gene.
What does the genetic code being non-overlapping mean?
-The genetic code being non-overlapping means that each triplet of bases (codon) is read individually without overlapping into the next triplet, ensuring a specific and consistent translation of the mRNA sequence into amino acids.
How did experiments with T4 phage contribute to understanding the genetic code?
-Experiments with T4 phage showed that mutations causing an addition or deletion of one or two bases resulted in nonfunctional proteins, while three base additions or deletions close together resulted in functional proteins with only small changes, indicating that the genetic code is read in triplets.
Outlines
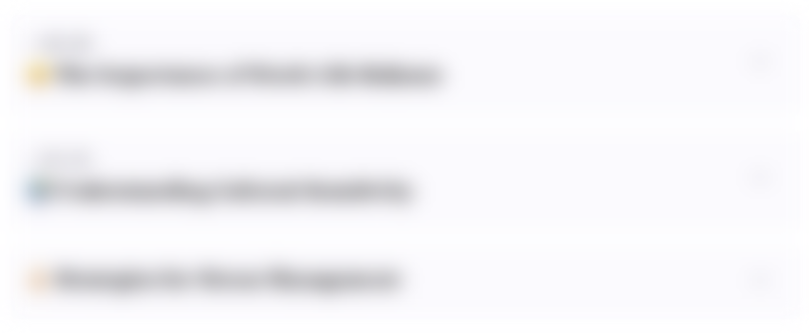
Dieser Bereich ist nur für Premium-Benutzer verfügbar. Bitte führen Sie ein Upgrade durch, um auf diesen Abschnitt zuzugreifen.
Upgrade durchführenMindmap
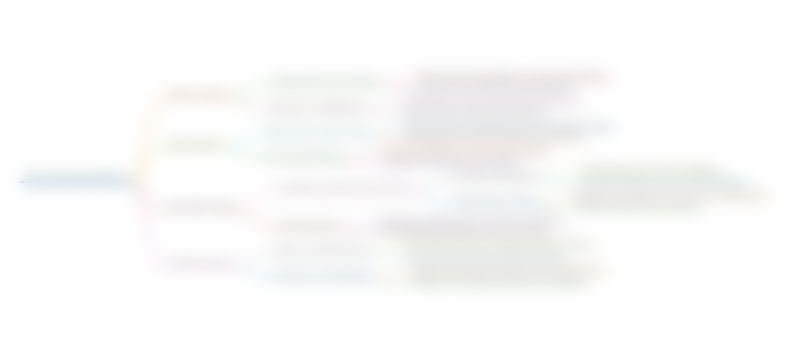
Dieser Bereich ist nur für Premium-Benutzer verfügbar. Bitte führen Sie ein Upgrade durch, um auf diesen Abschnitt zuzugreifen.
Upgrade durchführenKeywords
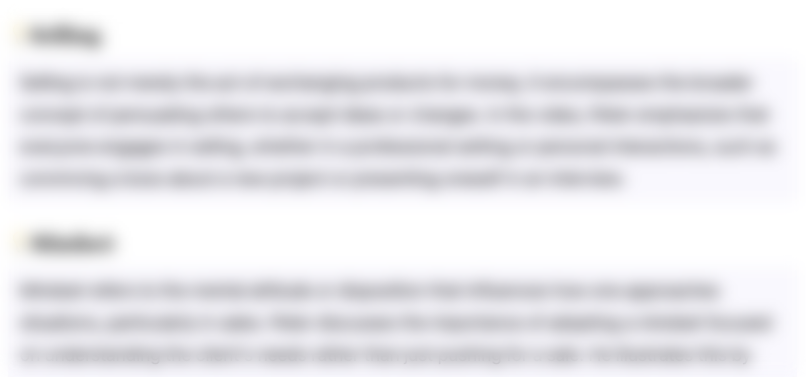
Dieser Bereich ist nur für Premium-Benutzer verfügbar. Bitte führen Sie ein Upgrade durch, um auf diesen Abschnitt zuzugreifen.
Upgrade durchführenHighlights
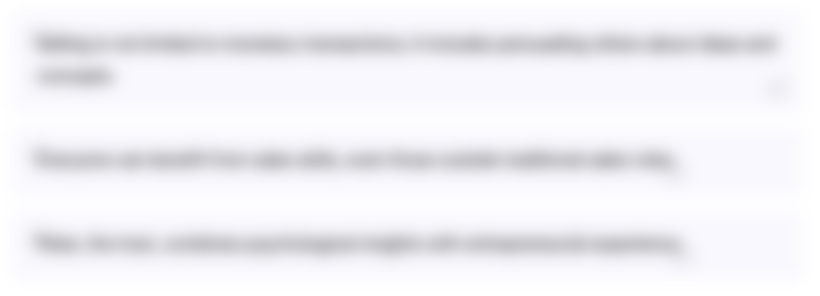
Dieser Bereich ist nur für Premium-Benutzer verfügbar. Bitte führen Sie ein Upgrade durch, um auf diesen Abschnitt zuzugreifen.
Upgrade durchführenTranscripts
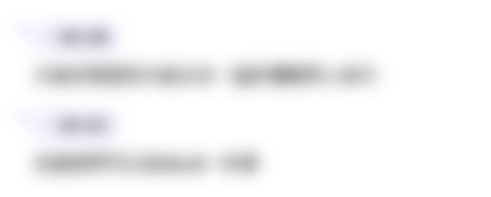
Dieser Bereich ist nur für Premium-Benutzer verfügbar. Bitte führen Sie ein Upgrade durch, um auf diesen Abschnitt zuzugreifen.
Upgrade durchführen5.0 / 5 (0 votes)