How lasers work - a thorough explanation
Summary
TLDRIn this informative video, Paul from Physics High Lasers explains the fundamental principles behind laser technology. He begins by highlighting the ubiquity of lasers in various industries and applications. The script delves into the atomic structure, the Bohr model, and the concept of discrete energy levels, leading to the explanation of why lasers are monochromatic, coherent, and collimated. Paul discusses the process of stimulated emission and the importance of achieving population inversion to generate a laser beam. The use of mirrors to create a standing wave and the resulting amplification of photons is also covered, culminating in the production of a focused, monochromatic beam of light.
Takeaways
- 🌈 Lasers are widely used in various fields such as industry, medicine, and everyday life like scanning groceries at supermarkets.
- 🔬 The acronym LASER stands for Light Amplification by the Stimulated Emission of Radiation, which is the fundamental principle behind how lasers work.
- 🎨 A laser beam is monochromatic, meaning it produces light of a single color due to the discrete energy levels of electrons in atoms.
- 🌊 The beam is coherent, with all the light waves being in phase, which is a result of the stimulated emission process.
- 📏 The collimated beam of a laser is very focused and travels in a single direction, which is essential for its applications.
- 💡 The Bohr model of the atom helps explain the discrete energy levels and the process of photon absorption and emission.
- 💥 The process of stimulated emission involves an electron absorbing a photon and then releasing two identical photons, leading to amplification.
- 🔝 Population inversion is a key concept where more electrons are in the excited state than the ground state, which is necessary for the laser effect.
- 🔄 The use of a metastable state allows electrons to stay in an excited state longer, increasing the chances of stimulated emission.
- 🔄 Mirrors are used in a laser setup to create a resonance effect, forming a standing wave that amplifies the photon population exponentially.
- 🚀 A partially transparent mirror allows the coherent laser beam to exit the laser cavity while reflecting most of the light back for further amplification.
Q & A
What are some common uses of lasers mentioned in the script?
-Lasers are used in various applications such as pointing at objects like wedding rings, in industrial applications, medical devices including eye surgery, and in supermarkets for scanning groceries.
What are the three main characteristics of a laser beam?
-The three main characteristics of a laser beam are that it is monochromatic (produces only one color), coherent (waves are in phase with each other), and collimated (a focused beam).
What does the acronym LASER stand for?
-LASER stands for Light Amplification by the Stimulated Emission of Radiation.
What is the Bohr model of the atom and why is it relevant to understanding lasers?
-The Bohr model of the atom is a simplistic model that depicts electrons existing in discrete orbits or energy levels. It is relevant to understanding lasers because the discrete energy levels determine the specific energy (and thus color) of the photons emitted, contributing to the laser's monochromaticity.
How does the energy difference between electron energy levels relate to the color of the laser light produced?
-The energy difference between electron energy levels corresponds to the energy of the photons produced, which determines the color of the laser light. Since the energy levels are discrete, the laser light is monochromatic, meaning it only produces one color.
What is the process of stimulated emission and how does it contribute to the coherence of a laser beam?
-Stimulated emission is a process where an electron in an excited state is stimulated by a photon, causing it to drop to a lower energy level and release two photons of the same energy, frequency, and phase. This process contributes to the coherence of a laser beam because it ensures that all photons are in phase with each other.
What is population inversion and why is it necessary for a laser to function?
-Population inversion is a condition where there are more electrons in the excited state than in the ground state. It is necessary for a laser to function because it increases the probability that a photon will stimulate the emission of additional photons, leading to a cascading effect that amplifies the light.
What is a metastable state and how does it help in achieving population inversion?
-A metastable state is an energy level that an electron can occupy for a relatively longer time compared to other excited states. It helps in achieving population inversion by allowing more electrons to stay in the excited state for a longer duration, increasing the likelihood of stimulated emission when a photon encounters these electrons.
How do mirrors play a role in the production of a laser beam?
-Mirrors are used to reflect photons back and forth within the laser medium, creating a standing wave and a resonance effect. This process amplifies the number of photons and ensures that the laser beam is coherent and collimated.
What is the significance of the standing wave in a laser and how does it contribute to the laser's properties?
-The standing wave is significant because it represents a resonance condition where the photons are amplified as they travel back and forth between the mirrors. This contributes to the laser's properties by ensuring a high level of coherence and a tightly focused, collimated beam.
How is the wavelength of the laser light determined in a laser setup?
-The wavelength of the laser light is determined by the length of the laser tube and the resonance condition set up by the standing wave. By adjusting the length of the tube, the right resonance for a specific wavelength can be achieved, producing a laser beam of that particular color.
Outlines
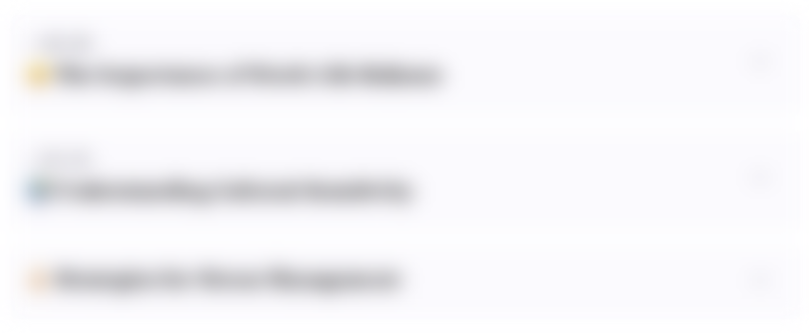
هذا القسم متوفر فقط للمشتركين. يرجى الترقية للوصول إلى هذه الميزة.
قم بالترقية الآنMindmap
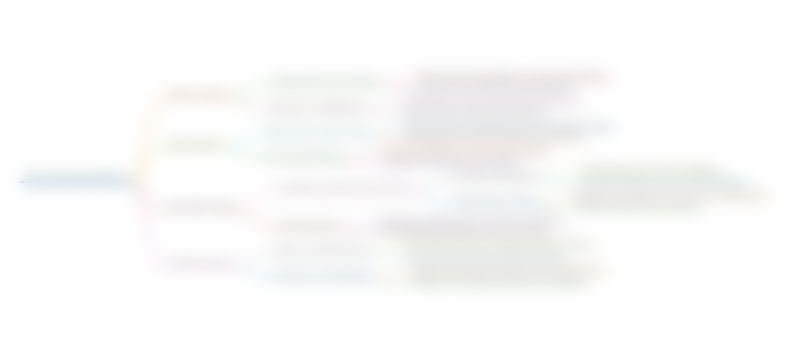
هذا القسم متوفر فقط للمشتركين. يرجى الترقية للوصول إلى هذه الميزة.
قم بالترقية الآنKeywords
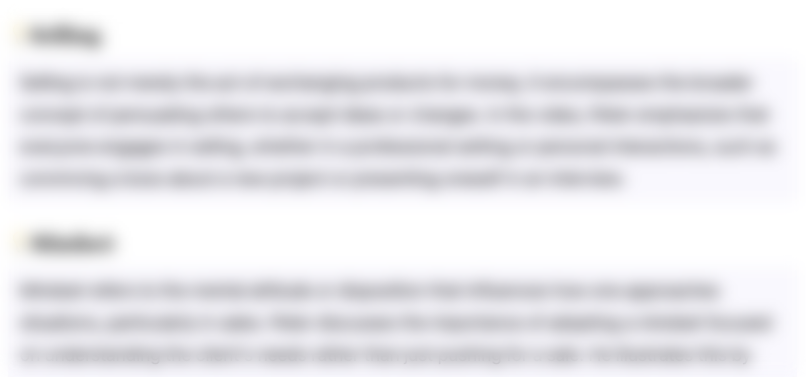
هذا القسم متوفر فقط للمشتركين. يرجى الترقية للوصول إلى هذه الميزة.
قم بالترقية الآنHighlights
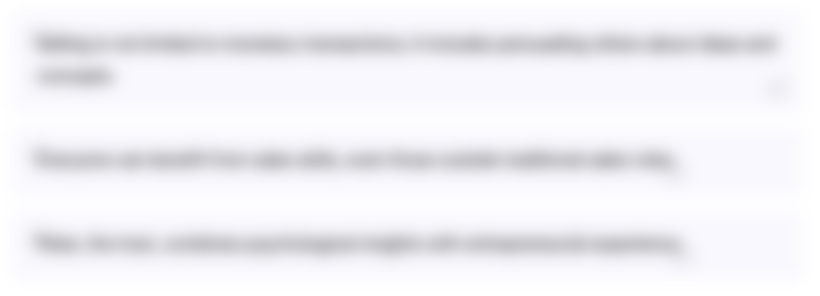
هذا القسم متوفر فقط للمشتركين. يرجى الترقية للوصول إلى هذه الميزة.
قم بالترقية الآنTranscripts
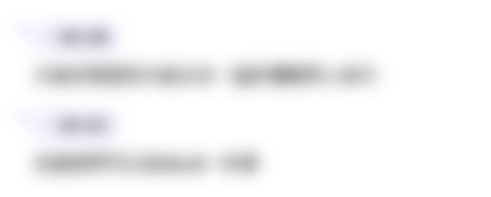
هذا القسم متوفر فقط للمشتركين. يرجى الترقية للوصول إلى هذه الميزة.
قم بالترقية الآن5.0 / 5 (0 votes)